This paper changed my life: ‘Histone demethylation mediated by the nuclear amine oxidase homolog LSD1,’ from the Shi Lab
This paper defined key rules of epigenomic regulation and shaped how I study chromatin plasticity as a mechanism for experience-dependent changes in the brain.
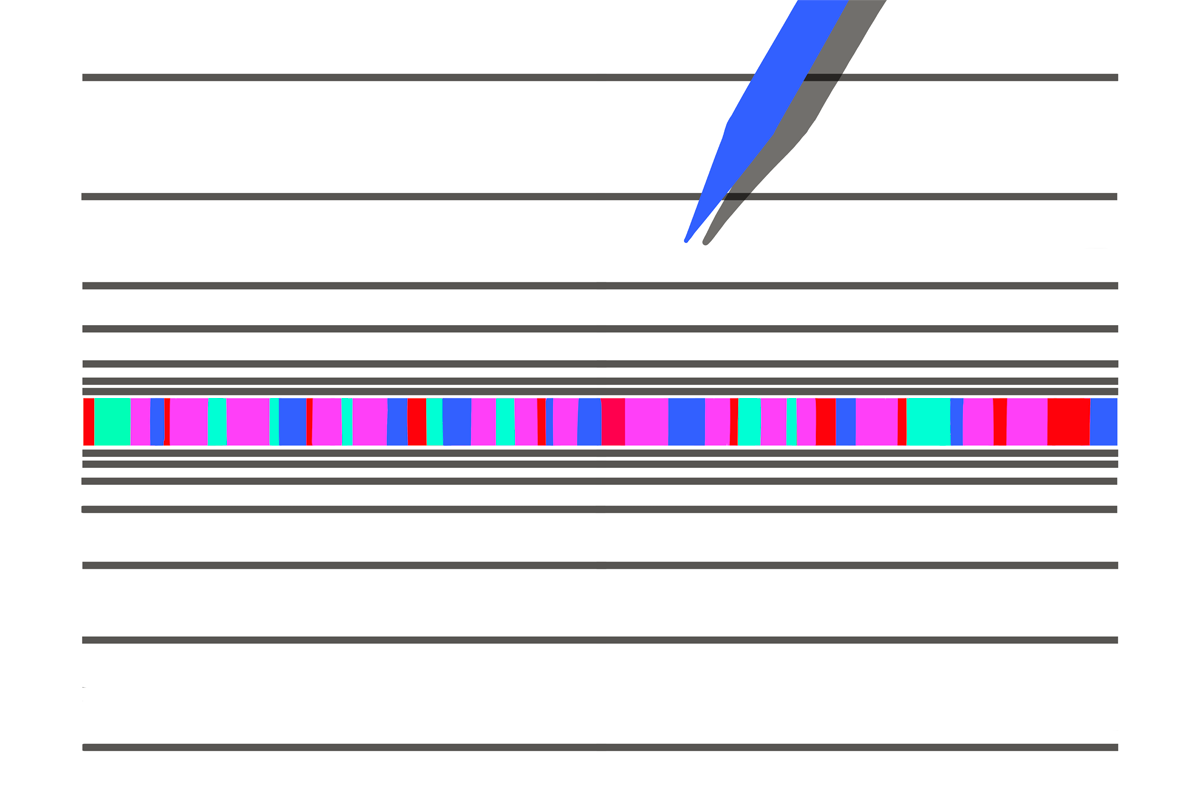
Answers have been edited for length and clarity.
What paper changed your life?
Histone demethylation mediated by the nuclear amine oxidase homolog LSD1. Shi Y., Lan F., Matson C., Mulligan P., Whetstine J.R., Cole P.A., Casero R.A. and Yang S. Cell (2004)
In this paper, Yang Shi and his colleagues reported the first histone demethylase—an enzyme that removes methyl groups from chromatin to regulate DNA transcription—which they named lysine-specific demethylase 1 (LSD1). They had previously isolated this protein as part of a transcriptional repressor complex that contained other histone-modifying enzymes, but the function of this component had been unknown.
Using a rigorous series of in-vitro approaches, the researchers found that LSD1 catalyzes the demethylation of lysine residues on histones in a site-specific manner. Then, using RNA inference and chromatin immunoprecipitation, they showed that LSD1-mediated demethylation of histone H3K4 repressed transcription of LSD1-bound target genes.
Methylation was considered a strong candidate to store memories in cells, so the discovery of a protein that could reverse this biochemical modification was of immediate interest to neuroscientists studying molecular mechanisms of brain plasticity.
When did you encounter this paper?
I heard Yang Shi present part of this story before publication at a seminar at Boston Children’s Hospital in 2003, when I was a postdoctoral researcher in Mike Greenberg’s lab. I still have my notes from that talk, where I drew stars and arrows around the part at the end when he teased the discovery of LSD1. I remember eagerly awaiting the rest of the story, and I am pretty sure that I read this paper on the day it was published in 2004.
Why is this paper meaningful to you?
During my postdoc, I was studying transcriptional regulatory mechanisms that mediate activity-dependent development of neurons. By the early 2000s, it was becoming clear that to understand this process we needed to move beyond thinking locally about transcription-factor binding at single-gene promoters and start working to understand how transcription was influenced by chromatin state and structure in the nucleus. This period was after the sequencing of the human genome, so we knew quite a bit about genes and gene products. But it was before genome-wide chromatin sequencing methods were adapted to mapping the epigenome.
The identification of histone-modifying proteins and the characterization of their exquisite site specificity described in this paper were essential for understanding the functional regulation of different types of histone modifications, which were hypothesized to form a “histone code.”
How did this research change how you think about neuroscience or challenge your previous assumptions?
At the time this paper came out, the epigenetics field was skeptical that histone or DNA methylation could be reversed once it was induced in cells. The more popular belief was that methylation was a nearly permanent mark on the genome, and this made it an intriguing candidate for cellular memory storage. However, if these chromatin marks could never be reversed, then they were unlikely to meaningfully contribute to the kinds of dynamic transcriptional adaptations that my postdoc lab and others were finding to underlie activity-dependent neuronal plasticity.
The discovery of the histone demethylases, which preceded the discovery of mechanisms for DNA demethylation, was a pivotal moment for recognizing the importance of this form of chromatin plasticity as a mechanism for experience-dependent changes in cellular function in the brain.
How did this research influence your career path?
My colleagues and I saw the potential of using histone-modifying enzymes to dissect functions of the epigenome in different kinds of neurobiological processes. I started by looking for activity-dependent regulation of the genes encoding these enzymes to identify members with possible functions in neural plasticity. These results led me to focus on KDM6B, a gene that encodes a lysine-27-specific histone demethylase.
Later research would show that mutations in several members of the histone demethylase gene families, including KDM6B, are associated with neurodevelopmental conditions, such as intellectual disability and autism. In response, I expanded our research program to develop mouse and human neuron models to discover mechanisms linking KDM6B’s regulation of chromatin with the changes in synapse and circuit function that underlie neurodevelopmental conditions.
It remains an open question whether different members of the histone demethylase family disrupt brain development through convergent or independent mechanisms, but the lysine site-specificity discovered in the original LSD1 paper remains a key conceptual guidepost for experimental approaches to this question.
Is there an underappreciated aspect of this paper that you think other neuroscientists should know about?
This paper shows that sometimes the most important contributions to a question in neuroscience come from a paper outside the field. What always impressed me about this study is how the detailed understanding of LSD1 biochemistry in vitro allowed for the discovery of epigenome dynamics that fundamentally changed the way we think about epigenetics in brain plasticity in vivo.
What paper changed your life?
Recommended reading
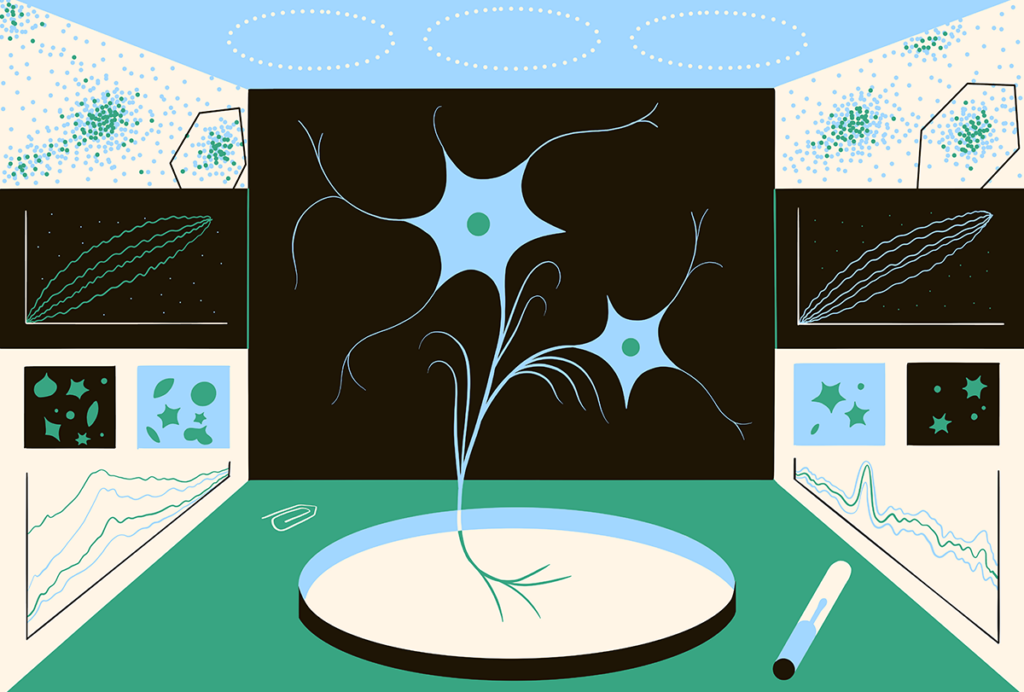
This paper changed my life: Shane Liddelow on two papers that upended astrocyte research
Explore more from The Transmitter
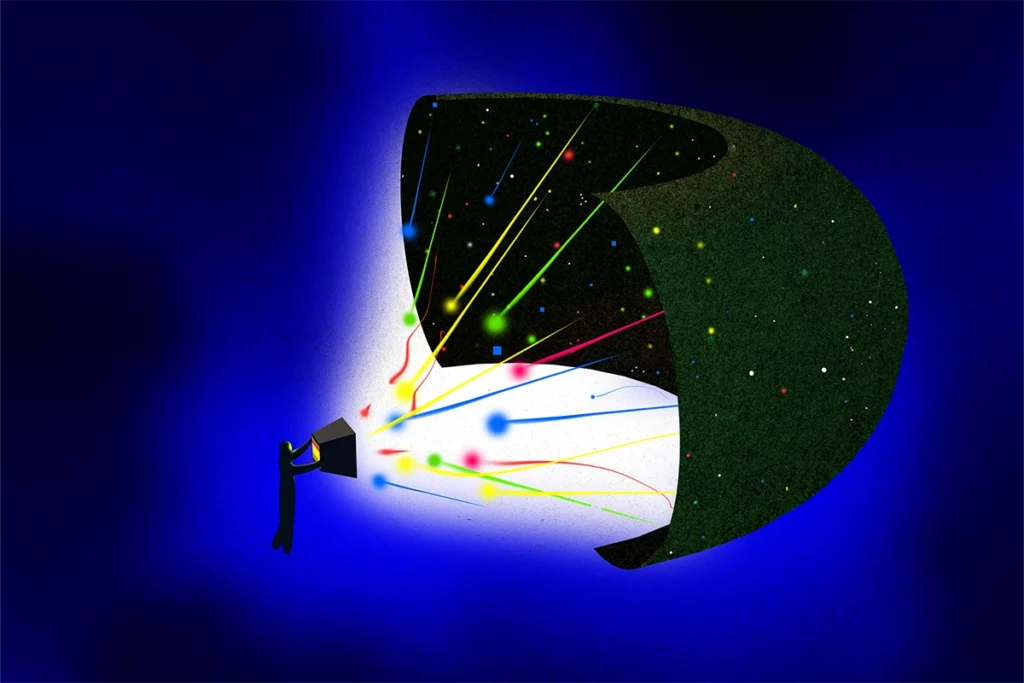
Imagining the ultimate systems neuroscience paper
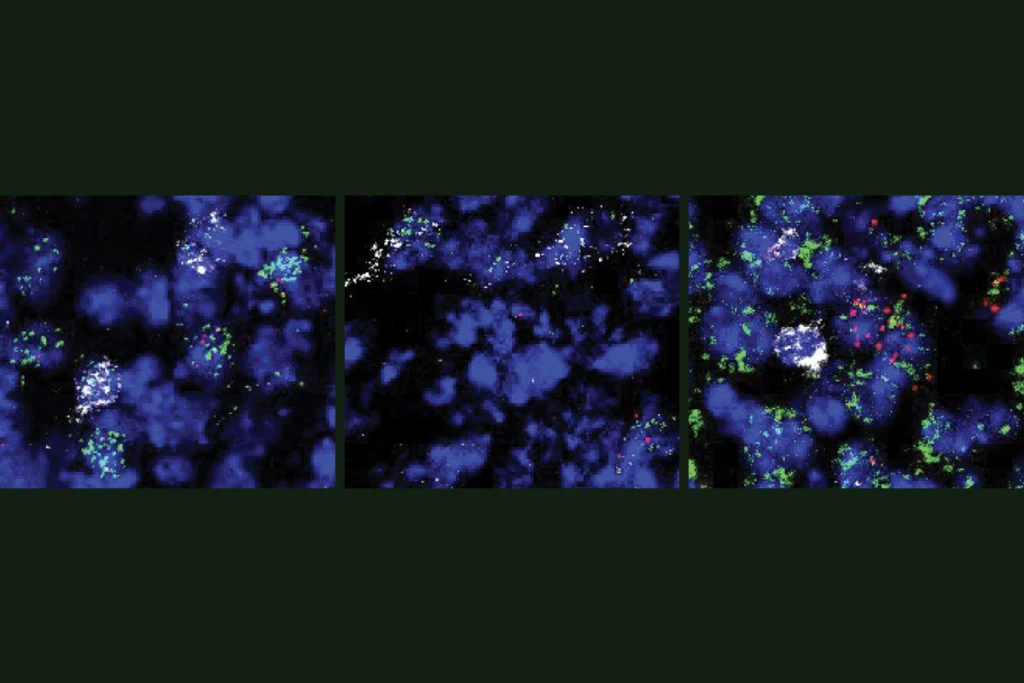
Novel neurons upend ‘yin-yang’ model of hunger, satiety in brain
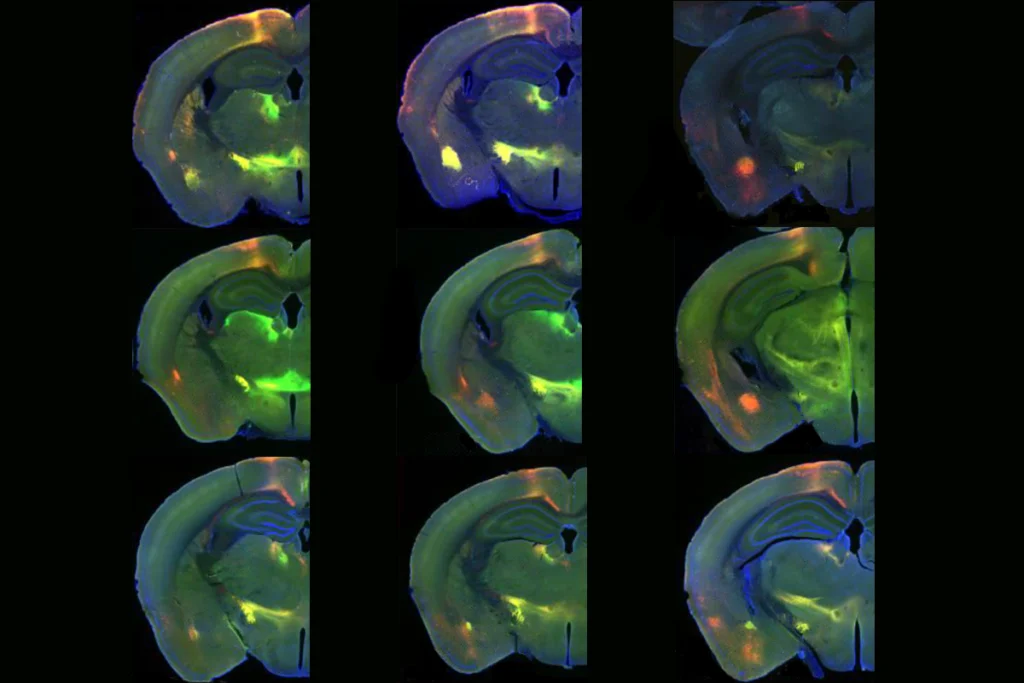