Trapping cells in droplets may bare basic autism mysteries
New, miniaturized single-cell sequencing techniques are helping researchers tease out how autism-linked genes work in the brain.
Now that researchers have linked hundreds of genes to autism, they face the tricky task of teasing out when and where these genes are expressed in the brain. Two new papers published in Cell last year revealed a powerful shortcut to profiling gene expression patterns that may propel autism research forward1,2. Their game-changing solution? Separating cells into microscopic droplets of water.
This new strategy offers many advantages over traditional approaches to measuring gene expression. One of these older methods measures gene expression in tiny chunks of postmortem brain tissue from individuals with autism. But chunks of tissue contain many cell types — a mixture that may mask important differences between individual cells.
An alternative is to pluck single cells from the tissue. But using traditional techniques, it would be impractical to individually analyze even a tiny fraction of the 100 billion neurons of the human brain, at a prohibitive cost of roughly $10 per cell.
Two research teams at Harvard University discovered that analyzing cells in tiny water droplets boosts the throughput and cuts the cost of the single-cell approach, allowing researchers to profile thousands of cells per day for as little as 7 cents each.
“To do the kinds of experiments we wanted to do, we believed that we were going to need a technology that was radically different,” says Steve McCarroll, associate professor of genetics at Harvard, who led one of the teams.
Dropping out:
McCarroll’s technique, described last May in Cell, is called ‘Drop-seq’1. The first step is to bathe tissue in an enzyme that breaks up the tissue’s protein scaffolding, separating it into a slurry of individual cells. The researchers then inject this water-based mixture into a narrow groove on a microfluidic chip, a thin glass wafer with tiny channels etched on its surface, causing the cells to flow in single file.
This stream of cells merges with a second watery stream containing microscopic plastic beads. Each bead is coated with short strands of DNA that bind to a cell’s messenger RNAs (mRNAs) and label them with a barcode that is unique to each cell.
A third channel containing oil then intersects this merged stream, separating it into tiny droplets. After days of careful calibration, the researchers optimized the flow rates and concentrations of cells and beads so that each droplet contains, at most, a single cell and a single bead.
Each cell breaks open within its droplet, and its mRNAs stick to the DNA strands on the bead. Researchers can then sequence the mRNAs — and the barcode they are labeled with — to determine the extent to which any gene is expressed in a given cell. The barcodes allow researchers to identify all of the mRNAs from a single cell.
Big benefits:
The miniscule droplets dramatically scale down the volume in which researchers sequence mRNAs, slashing costs. And the vast number of droplets the technique creates results in an enormous boost in efficiency: Researchers can profile roughly 10,000 cells in 12 hours.
“Those two things allow you to do lots [of cells] and allow you to do it cheaply,” says Ed Lein, investigator of human cell types at the Allen Institute in Seattle, Washington, who was not involved in the work.
Around the same time, another team at Harvard, led by physicist David Weitz and cell biologist Marc Kirschner, developed a similar approach, called inDrop2. The two techniques use different types of beads and different means of making droplets, but achieve comparable results. Drop-seq generates droplets quickly, but few of them contain both a bead and a cell. By contrast, inDrop makes droplets at a slower rate, but more of them house both components.
Either approach provides a snapshot of the genes that are turned on or off in a cell. Researchers can then feed individual cell profiles into a computer program that reveals patterns of gene expression among a range of different cells in a tissue. For example, McCarroll’s team used Drop-seq to profile nearly 45,000 cells from mouse retinas, which are thought to harbor about 100 different cell types. They identified only 39 distinct gene expression profiles, however, suggesting fewer cell types.
“Now we have an extremely rich, quantitative way of just trying to tell what the cell types are,” says Lein. “This measurement, in one fell swoop, recapitulates most of what the entire field has found with a collective effort over decades.”
Brain atlas:
McCarroll’s team plans to apply the approach to entire mouse brains.
“We’re getting enough profiles from individual cells so that we can start to create an atlas of cell types in the brain,” says Evan Macosko, a postdoctoral fellow in McCarroll’s lab who helped to develop Drop-seq.
Such an atlas would be a powerful tool for neuroscientists, says Jason Stein, assistant professor of genetics at the University of North Carolina at Chapel Hill, because no one knows for sure how many cell types make up the brain. Estimates range from dozens to even hundreds. “Having a parts list will hopefully help us better understand how the brain works,” Stein says.
McCarroll’s team is also using single-cell sequencing to understand how autism-linked gene variants affect gene expression in specific brain cell types in mice. Along with Beth Stevens, assistant professor of neurology at Harvard, the group is investigating how autism-linked variants may alter gene expression in immune cells called microglia during brain development.
“I think Drop-seq could offer very helpful ways to study mouse mutants and ask what’s really wrong with them,” McCarroll says.
Tissue tool:
Drop-seq may also be able to clarify results from certain studies probing brain tissue from people with autism. For example, a 2011 study of gene expression patterns in brain tissue from people with autism hinted that microglia and another brain cell type called astrocytes may be altered in people with the condition. A similar study published in 2014 also implicated microglia.
But because these findings stemmed from mixtures of cells, they may simply reflect alterations in the numbers of certain cell types rather than signaling true changes in gene expression. Using Drop-seq, researchers could zero in on the cell types that have altered gene expression.
Yet there is one major obstacle: The method requires intact cells. Postmortem brain tissue from people is typically frozen and then thawed for research — a process that bursts cell membranes.
“The fundamental idea of the approach is that it uses an intact plasma membrane to be able to tell what RNA came from what cell,” McCarroll says.
Obtaining brain tissue from people with autism undergoing brain surgery for, say, epilepsy or brain cancer, circumvents this problem. But opportunities for collecting fresh surgical tissue are rare, and all such individuals would have a brain disorder aside from autism, complicating the findings.
To make droplet-based approaches work with postmortem tissue, McCarroll’s team is modifying the Drop-seq protocol to work with individual cell nuclei, whose membranes withstand the freeze-thaw process. The nucleus contains fewer mRNAs than the cytoplasm, but the overall expression profile there is similar to that in the cell’s main compartment.
Researchers imagine other useful tweaks. On the wish list of Eric Courchesne, professor of neuroscience at the University of California, San Diego, is the ability to determine a cell’s DNA sequence as well its RNA expression profile. The sequence information could clarify the effects of ‘somatic’ mutations — spontaneous genetic changes that strike only a small subset of a person’s cells.
In a 2014 study, Courchesne and his colleagues found small patches of cells that may have migrated to the wrong brain regions in some people with autism. If cells in these patches have unique DNA sequences and RNA expression patterns, that would tell us a great deal about the processes that led to these patches, he says.
Drop kick:
One limitation of both Drop-seq and inDrop is that they favor genes expressed at high levels. “We get cell-specific information, but we capture primarily the abundant transcripts,” says Irina Voineagu, senior lecturer of biological sciences at the University of New South Wales in Sydney, Australia.
And neither method can identify ‘splice variants’ — slightly different copies of the same gene created by mixing and matching different DNA segments. Several studies hint that splicing may go awry in autism.
Still, the popularity of these techniques speaks volumes about their potential. The McCarroll lab has posted a 20-page, illustrated instruction manual for Drop-seq. “It’s been downloaded from our website more than 3,000 times,” McCarroll says. “And we’re corresponding with hundreds of groups that either have set up, or are setting up, or are considering setting up the technology in their own labs.”
Meanwhile, the researchers who developed inDrop are commercializing their approach. They are launching a company that plans to sell kits containing a microfluidic chip, beads and other reagents. Weitz says the company, called One Cell, should be open for business in a few months.
The techniques could help autism researchers crack the molecular ‘code’ that links gene sequence and expression patterns to cell function, and ultimately to behavior, Lein says. “I honestly feel that we’re on the cusp of a transformation in this field.”
References:
Recommended reading
Assembloids illuminate circuit-level changes linked to autism, neurodevelopment
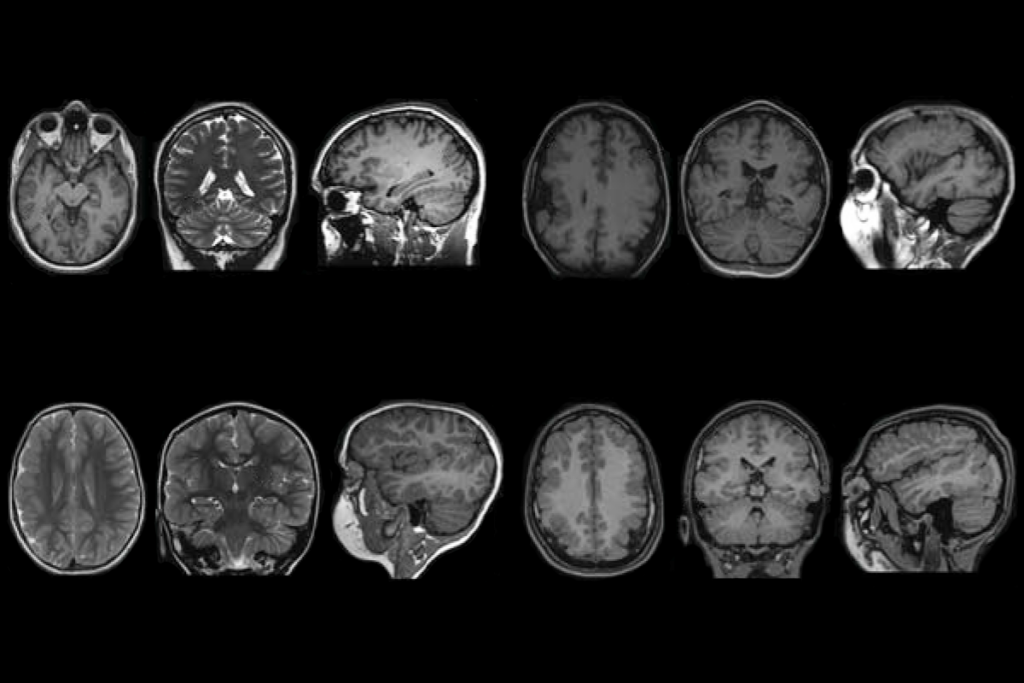
Impaired molecular ‘chaperone’ accompanies multiple brain changes, conditions
Explore more from The Transmitter
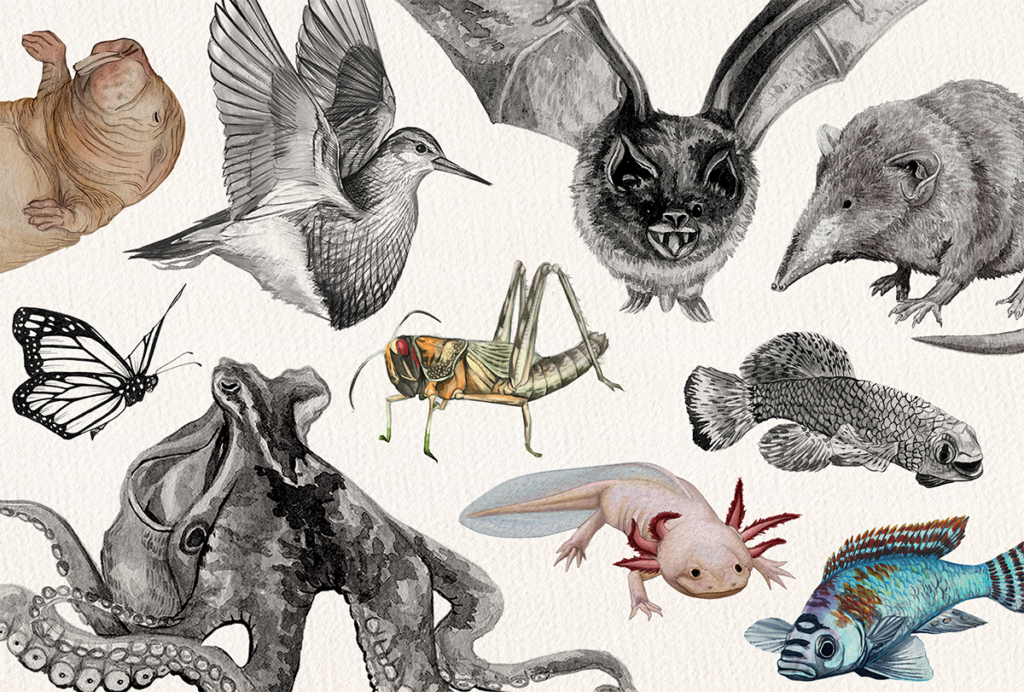
The non-model organism “renaissance” has arrived
Rajesh Rao reflects on predictive brains, neural interfaces and the future of human intelligence
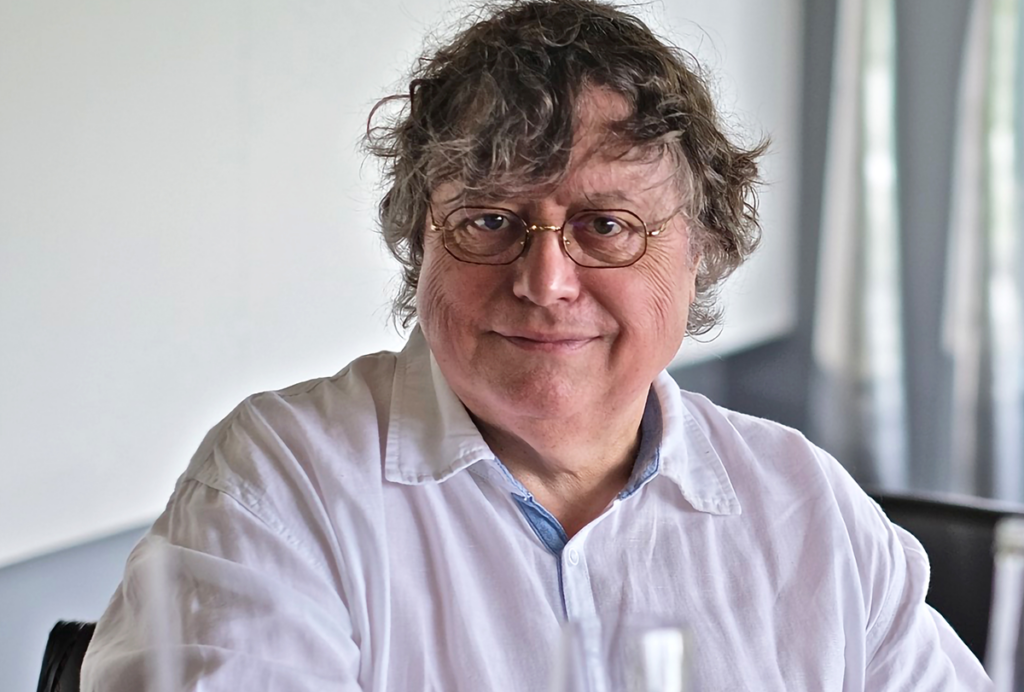