The brain’s secret gardeners
Once thought merely to be specialized immune system cells, microglia now appear to be master landscapers of the developing brain.
The brain of a newborn baby is an overgrown garden. Billions of neurons connect via an unimaginable number of junctions, or synapses, far more than will remain in adulthood. For this thicket of connections to mature into a properly organized adult brain, a group of special immune cells called microglia must get to work. They pick their way among the overgrowth, shape-shifting the tendrils of their branch-like arms into blobs that rake up debris and damaged cells. They battle microbial invaders, engulfing their remains, and capture and destroy incorrectly folded proteins.
While they tend and clean, they also perform what may be their most important duty, and one scientists have recognized only over the past four years: They snip away synapses, a process that is essential for the brain to develop just the way it should. The activities of these tiny cells in the infant brain may have powerful and permanent effects on how the brain is wired, and on behavior far down the line.
Scientists are trying to untangle not only how microglia function in healthy brains, but also what they may be doing in the brains of people with autism, and whether they present a possible path to new treatments. Do microglia become active in response to physical damage, or some kind of genetically predisposed altered wiring? Or are dysfunctional microglia themselves the source of the trouble? These are some of the thorniest and most important questions facing the field, says neuroscientist Beth Stevens of Boston Children’s Hospital.
“The fundamental question is whether they’re contributing in a direct way, or just responding to other problems,” she says. “It’s totally ‘chicken or egg’ — which came first?”
First clues:
Microglia are immune cells, so for a long time scientists treated them only as such, paying little attention to their other activities. Along with seeking out and destroying invaders and the cells infected by them, microglia turn on in response to traumatic brain injury to gobble up injured cells, and become active in brains beset by multiple sclerosis or other disorders. Though they make up about 10 percent of the brain by cell count, most research on microglia focused on those limited functions, says neuroscientist Dori Schafer, who in 2014 left Stevens’ team to launch her own lab at the University of Massachusetts Medical School. “The tone of the research was that they are kind of quiescent in healthy, steady-state conditions, and their main job is to respond to injury and disease,” she says.
Signs that microglia are much more than ‘brain cleaners’ came into view in 2005, when the then-emerging technique of two-photon microscopy allowed two teams of researchers to watch the cells in action in living mouse brains and produce detailed images. Axel Nimmerjahn, then a graduate student at the Max Planck Institute for Medical Research in Heidelberg, Germany, captured movies of surprisingly active, mobile microglia. Another group, led by Dimitrios Davalos, then at New York University, caught microglia in action just before and during a traumatic brain injury. Far from sedentary sentinels just waiting for an injury to happen, his team found, they are in fact the fastest-moving cells in healthy adult brains.
Five years after the live imaging work came another major clue that microglia are more than mere immune responders. Miriam Merad and her colleagues at the Icahn School of Medicine at Mount Sinai in New York established that microglia originate just a few days after conception, far earlier in embryonic development than scientists had thought. That’s long before the emergence of any other cell-destroying mechanism, and offers a clue that these cells may be especially important for development, and not just for staving off diseases, says Stevens.
In fact, the evidence was starting to suggest that the cells might be involved in one of the most important processes in brain development: the careful pruning away of unnecessary or extraneous synapses in early infancy and childhood. Researchers now know that in both mice and people, sensory inputs trigger this process; as the newborn creature explores its world, cellular mechanisms in its brain respond, snipping away the connections that aren’t being used and strengthening those that are. Just as judicious trimming can cultivate the growth of a tree by directing its energies into strengthening a few strong branches, synaptic pruning helps neurons, their branches and the remaining synapses flourish.
With the new understanding of microglia’s active nature and early genesis, scientists suspected that the cells might be involved here, too. “Why else would they waste so much energy moving around?” asks Cornelius Gross, a biologist at the European Molecular Biology Laboratory in Monterotondo, Italy.
Indeed, in 2012, Stevens and Schafer found evidence that microglia do in fact shape the developing mouse brain right from birth, engulfing some synapses, especially those that are weak or less active than average. “It was a beautiful demonstration that microglia have a normal function during development, particularly in modeling of areas of the cerebral cortex and synaptic connectivity,” says Carlos Pardo-Villamizar, associate professor of neurology and pathology at Johns Hopkins University in Baltimore. These findings suggested another tantalizing idea: Microglia might actually be involved in all kinds of neurological conditions, from Huntington’s and Alzheimer’s diseases to autism.
In 2005, Pardo-Villamizar and his colleagues examined postmortem brain tissue from 11 people with autism who had died in accidents, and cerebrospinal fluid from 6 living people with autism. Their analysis found signs of inflammation in both sets of autism brains compared with the brains of controls, including spikes in microglia activity and proteins related to microglia. It was the first documentation of abnormal microglial activity associated with autism, Pardo-Villamizar says. His theory is that the cells respond to malformed connections in the cerebral cortex.
It’s complicated:
Like any relationship, the link between microglia and autism is complicated. Different experiments, some from the same labs, have yielded seemingly contradictory findings. In some studies of certain mouse models of autism, getting rid of microglia improves symptoms seen in some people with autism-related disorders, such as breathing and movement problems; other studies have found the opposite.
For example, Jonathan Kipnis’ team at the University of Virginia in Charlottesville studied mice lacking a gene called MeCP2, the predominant cause of Rett syndrome, an autism-related disorder that primarily affects girls. Replacing the mice’s microglia via a bone marrow transplant improved their abnormal breathing patterns and apnea, symptoms also found in children with Rett. But follow-up studies at different labs, using the same methods, found no benefit. Schafer has also been studying mice missing MeCP2, and her unpublished findings show that microglia’s cell-eating capability increases in later stages of the syndrome. She says this suggests the cells are playing a clean-up role in the brain.
But the evidence doesn’t all point in this direction: If microglia are crucial, problems with the cells would be expected to have big effects in the brain. This doesn’t always seem to be the case. In a 2014 study by a team at the University of California, Irvine, otherwise healthy mice were given a drug that depletes up to 99 percent of their microglia, and researchers saw no demonstrable effect on behavior.
“We don’t know what [microglia] do,” says Anthony Filiano, a postdoctoral researcher in Kipnis’ lab. “It’s really surprising that you would be able to get rid of these cells and have no obvious deficits.”
To come to grips with these seemingly contradictory results, scientists are trying to decode the cross talk between microglia and other cells. In the first few weeks after birth, baby mice produce a protein called fractalkine, which communicates with microglia and seems to be needed for pruning synapses. Expression of this protein ramps up dramatically in the second week after birth, and the protein binds to a receptor found only on microglia. When Gross’ team bred mice in 2011 lacking the receptor protein, the mice wound up with more synapses than usual, and the excess synapses persisted into adulthood.
What’s more, the synapses were weaker than in controls; the neurons in the mice’s prefrontal cortex lacked what’s called synaptic ‘multiplicity’ — the process by which two neurons initially connect through a single synapse and add more connections over time to strengthen their bond. “Presumably, this is important for the strength and efficacy of the synaptic inputs,” says Gross. The multiplicity deficit changed the mice’s behavior; they spent more time grooming themselves and avoided social contact — behaviors that are taken to represent autism symptoms in mice.
The possibility that problems with microglia affect connectivity in the prefrontal cortex is intriguing, Gross says. Many studies have linked autism to abnormal functional connectivity (the coordinated activity between brain regions that are physically separate from each other).
“In the autism literature, people have talked about weak functional connectivity, but nobody has proposed specific synaptic mechanisms that might underlie that,” Gross says. The weak synaptic multiplicity that his group found could explain this phenomenon — and microglia are squarely implicated.
Ralph-Axel Müller, who directs the Brain Development Imaging Laboratory at San Diego State University, cautions that the experiments are intriguing but far from a final answer. “There are so many different lines of research that seem to tell us something about autism spectrum disorders, and microglia is certainly one of them,” Müller says. “But [autism] is not one disorder. So it’s possible that microglia are affected in some subtypes of the disorder; it’s very unlikely that this is somehow the silver bullet, or leads to a unique explanation of what happens in development.”
”“One of the cool things about these cells is that they’re constantly moving.” Dori Schafer
Illuminating results:
Amid the debate, there is one consensus: There needs to be more basic science on the secret nature of microglia.
“We don’t know what they do in the healthy brain, so it’s hard to understand what they’re doing in the pathological brain,” Filiano says. And that is likely to be a challenge. The cells recall a strange property of quantum mechanics known as Heisenberg’s uncertainty principle, in that the very act of observing the cells changes their status. Because the cells respond to injury, any little perturbation in the system can change the way they move, or affect which genes are activated and expressed.
Besides, while mouse models may be instructive, they are only approximations when it comes to brain development. Pruning and maturation of synapses occur at different times in men and mice, and the molecular correlates naturally vary as well.
Still, molecular research offers new possibilities for understanding microglia’s role, particularly in autism. Several teams are looking for genetic markers, or listening in to the chemical chatter between cells that leads to microglial activation. At the University of California, Los Angeles, Daniel Geschwind’s lab has evidence that microglia-related genes in people with autism are abnormally regulated. For example, the team found that the expression of genes related to synapse development is diminished, whereas that of genes involved in inflammation is enhanced. But Stevens notes that it’s not clear how microglia are involved in these changes, and whether they’re causing the alterations or responding to them.
In the meantime, Schafer is probing the mechanisms that underlie microglia’s ability to engulf weak synapses. In a typical experiment, her team sequesters a newborn mouse in total darkness. Without light, the development that would normally take place in the visual cortex during the first days of life is on hold. Then, on Day 5, the researchers switch on the lights for three hours and measure the rapid changes in gene expression that follow — changes that presumably drive the rapid remodeling and editing of synapses.
They dissect the mouse’s brain and remove the visual cortex, isolating the microglia in an attempt to find out what the cells were doing at that moment. They then compare these cells with those taken from mice raised in darkness with zero light exposure, as well as mice raised in a normal daily cycle of light and dark. The idea is to determine how changes in microglial gene expression cause the cells to snap into action, tearing synapses apart and remodeling the neural architecture.
Already, Schafer’s team has found that exposure to light changes microglial gene expression, and that the cells alter their movement in response to shifts in neuronal activity. It’s clear that neurons exposed to sudden changes are sending signals to microglia, but it’s not yet certain what these signals are or how they’re communicated, Schafer says. By establishing exactly which molecules spur microglia into action when the brain needs rewiring, her group may begin to understand how those molecules affect the brain’s architecture and neurons’ behavior — and ultimately, behavior in the animal.
Clearer pictures:
It is possible that microglia’s role in brain development extends even beyond pruning. As synapses form, genes related to microglia switch on, Gross notes, suggesting that the cells are involved in forming synapses, not just in destroying them. Stevens says microglia may be modulating the wiring of the brain, as well as regulating the development of newborn neurons. “I would say that pruning is probably the tip of the iceberg,” Stevens says.
Other scientists are studying the cells on a macro scale, improving imaging techniques that make it possible to observe microglia in their natural state. Physical observation has already yielded some of the best evidence that microglia actively prune synapses: Improved microscope techniques have allowed researchers to see signs of synapse detritus inside microglia’s cell-eating compartments. “What’s the nature of this synaptic material that we think is inside?” asks Gross. “That’s an active area of research now.”
The next phase is capturing microglia at work in awake, live animals. Schafer says several labs are adopting imaging techniques used for some time to observe astrocytes and neurons, which involves replacing a fragment of skull with a clear glass window over the brain. The mouse’s head is fixed in place while it runs on a wheel, and fluorescent protein tags allow scientists to watch microglia in action with unprecedented detail. “One of the cool things about these cells is that they’re constantly moving. You can’t really see that in a dissection of the brain,” Schafer says. “You get pretty big differences in cellular responses when you image them in an awake, behaving mouse versus an anesthetized mouse.”
Gross spent a year and a half developing his own new mouse line with microglia that light up under fluorescent microscopes, enabling him to capture images of active microglia a couple of times per minute. He is also exploring ways to harvest and culture small slices of brain tissue, potentially eliminating some of the challenges of scanning live brains, such as toxic effects from the light used to view cells. Since Nimmerjahn’s group debuted the two-photon movies, several teams have produced higher-resolution images of microglia dynamics in live mouse brains. Just last year, researchers reported an adaptive optics technique that can view microglia activity through a live mouse’s intact skull. Some teams are using the retina — technically part of the central nervous system, yet one that scientists can scrutinize in live animals without having to breach the skull — for visualizing microglia activation.
These approaches, along with new chemical analysis, may help scientists see how the brain’s gardeners tend the landscape of the brain. Understanding how the growth becomes too crowded, or too weak, could point the way toward microglia-related treatments for brain-related conditions, including autism, Stevens says.
“None of these studies thus far, in my opinion, address in a direct way whether they might be contributing to autism; that’s where we have to go,” she says. Five years ago, she says, researchers didn’t have the tools to genetically manipulate microglia or autism candidate genes — but they do now. “I think the time is right to address these questions.”
Corrections
This article has been modified from the original. Dori Schafer is at the University of Massachusetts Medical School, not the University of Massachusetts Amherst as previously stated.
Recommended reading
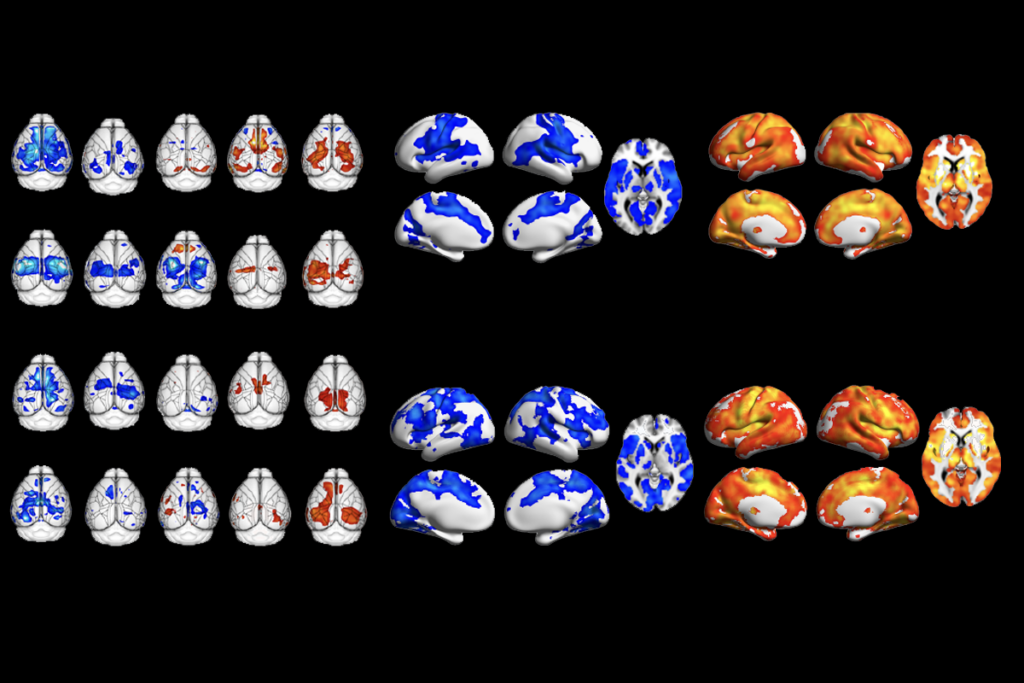
Too much or too little brain synchrony may underlie autism subtypes
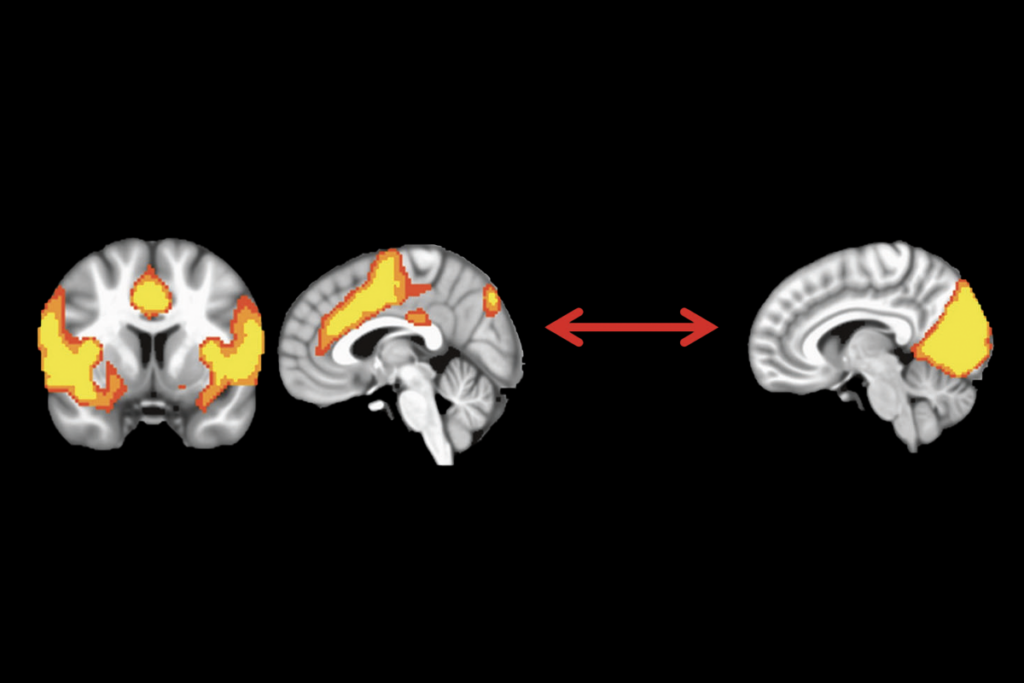
Developmental delay patterns differ with diagnosis; and more
Explore more from The Transmitter
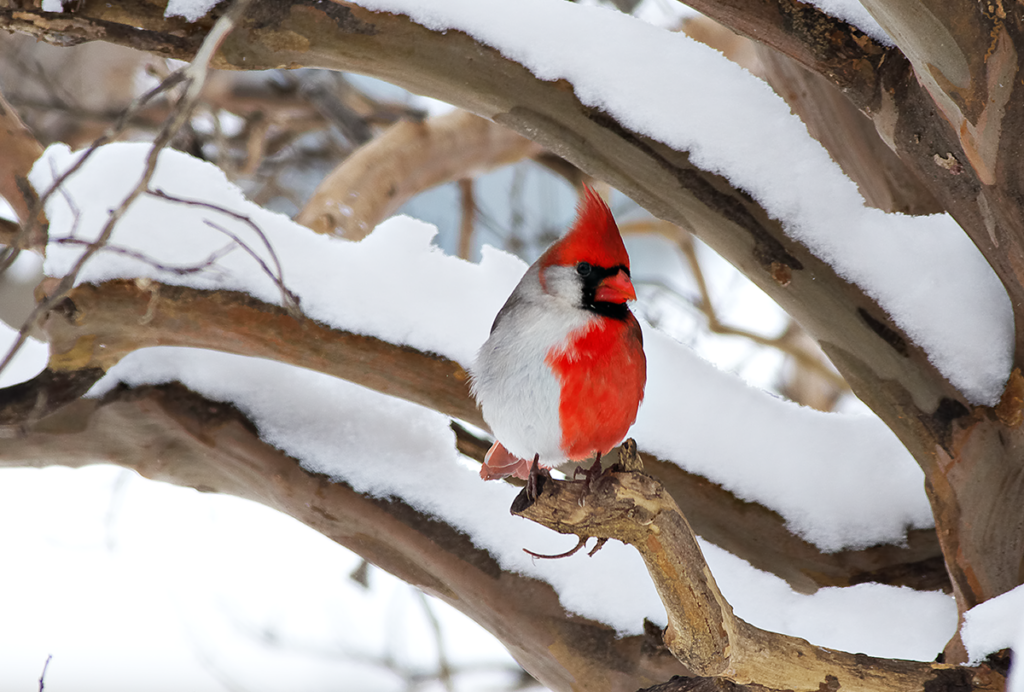
What birds can teach us about the ‘biological truth’ of sex
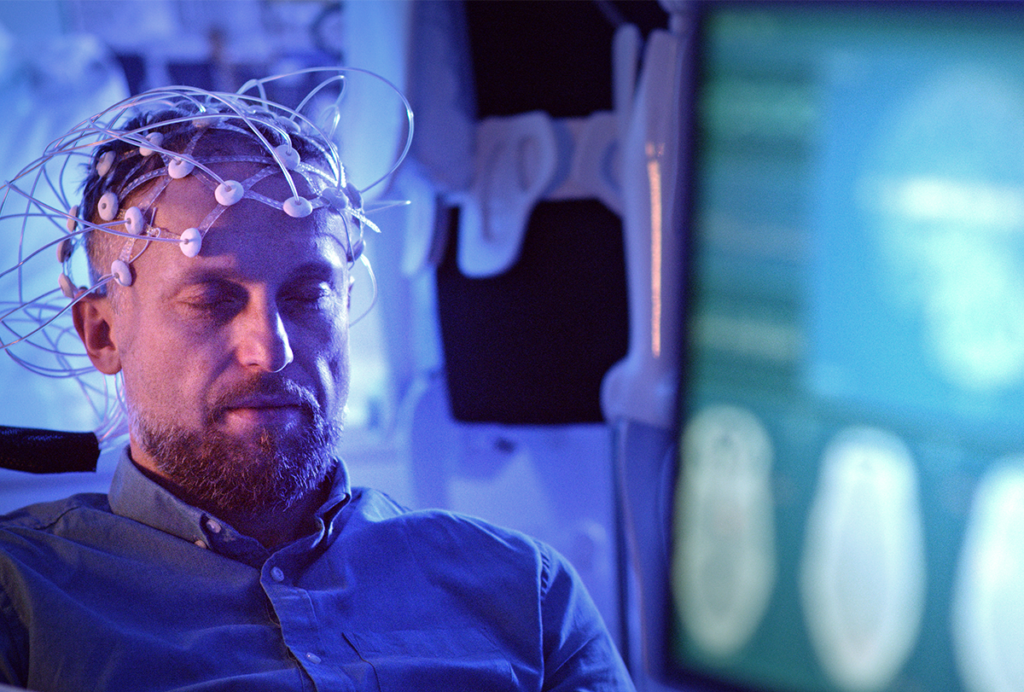
Noninvasive technologies can map and target human brain with unprecedented precision
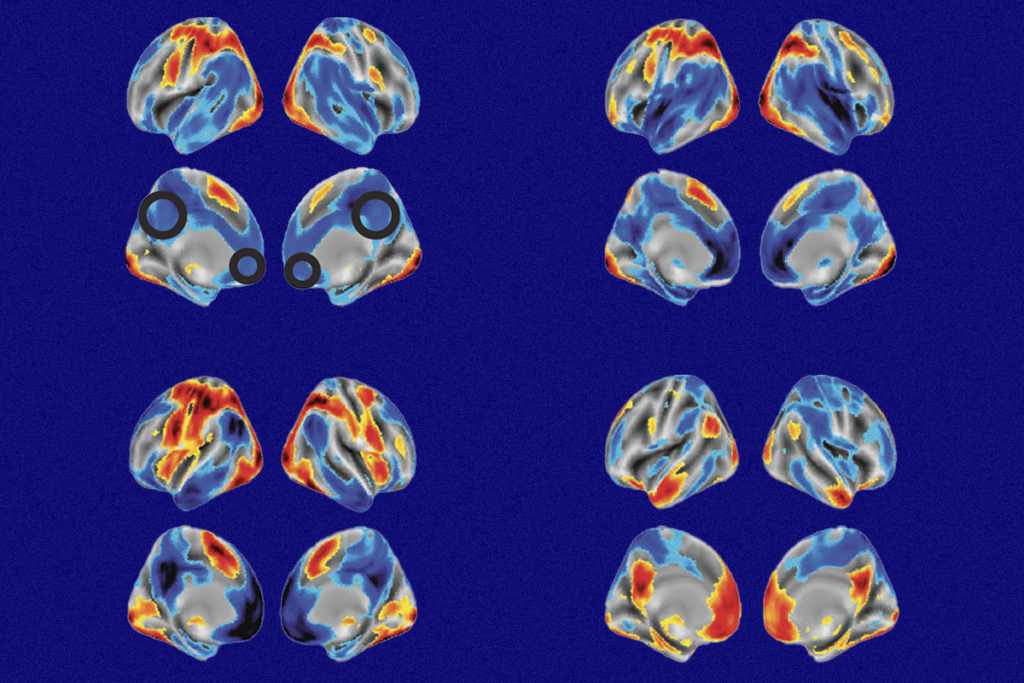