The 1999 Rett syndrome paper
Huda Zoghbi and her colleagues painstakingly sequenced the candidate genes for Rett syndrome, culminating in the 1999 Nature Genetics report that pinpointed six de novo mutations in the MeCP2 gene as the cause of the disorder.
In 1997 I took part in a workshop convened by the US National Institutes of Health to sift through the conflicting literature surrounding a rare and then poorly understood disorder known as Rett syndrome (RTT). Although the disorder was first described in Germany as early as the mid-1960s by Andreas Rett, it was not widely appreciated until 1983, when Bengt Hagberg and colleagues reported on a series of similar patients1,2.
RTT was quite intriguing, as it seemed to affect only females who appeared normal until about the age of one year, at which point they began a gradual loss of cognitive and motor skills that led ultimately to a complete loss of language and social interactions. Even in 1997, RTT remained largely mysterious, and many of us saw our first patient at the NIH workshop.
One of the overarching questions we sought to answer then was whether RTT is genetic. The syndrome consists for the most part of sporadic female cases and a handful of familial cases with either mildly affected mothers or two affected female siblings. Despite the absence of clear familial transmission and a troubling discordant monozygous twin pair, the consensus was that if RTT was in fact genetic, it was likely to be X-linked with lethality in hemizygous males.
Although such underwhelming evidence might deter other researchers from a full-blown gene hunt, Huda Zoghbi, one of the meeting’s organizers, had been fascinated by the syndrome ever since she had seen her first patient many years earlier. She, along with a couple of other groups ― most notably Stanford University’s Uta Francke ― took up the challenge.
Gene hunt
There were not enough RTT families for a traditional linkage analysis that could pinpoint a responsible gene on the X chromosome, but the Zoghbi and Francke laboratories, working both independently and collaboratively, performed exclusion mapping, in which large regions of the X chromosome where the gene would be unlikely to exist are ruled out.
By the end of the following year, it became clear that only one region of the X chromosome remained to be excluded: the terminal band of the long arm, Xq283. Unfortunately, at that time Xq28 was considered one of the most gene-dense regions in the human genome. Still, the undaunted investigators painstakingly sequenced the candidate genes, culminating in the 1999 Nature Genetics report that pinpointed six de novo mutations (four missense, one nonsense, and one frameshift) in the MeCP2 gene as RTT’s cause.
What’s more, in one of the RTT families with two affected half-siblings born to an unaffected mother, a missense mutation, similar to one in a sporadic case, was found in both half-sibs but not the mother, suggesting gonadal mosaicism in the mother.
The evidence was suddenly overwhelming: RTT is genetic, X-linked, and due in many cases to mutations in the MeCP2 gene.
The identification of MeCP2 was a watershed moment for RTT, immediately explaining many of its puzzling features. Because MeCP2 encodes a well-known protein involved in the epigenetic regulation of gene expression, its involvement also provided great insight into the pathophysiology of RTT.
We now know that 95 percent of RTT cases are a result of MeCP2 mutations, nearly all sporadic, mostly because of paternal germline mutations. Although more than 300 different MeCP2 mutations have been identified, only 8 missense and nonsense mutations account for up to about 70 percent of RTT mutations.
The vast majority of familial cases are a result of either gonadal mosaicism or skewed X-inactivation favoring the normal X chromosome, which leads to asymptomatic or mildly affected mothers. Surprisingly, the phenotypic spectrum of RTT dramatically broadened with the advent of MeCP2 genetic testing. In particular, the discovery of males with MeCP2 mutations has undercut the belief that RTT is exclusively female.
Since the 1999 publication, a number of males with MeCP2 mutations have been identified exhibiting a range of phenotypes. Some mutations that are penetrant in female RTT patients can lead to severe encephalopathy with neonatal death in males, but more subtle MeCP2 mutations, which do not cause RTT in females, can in males lead to moderate mental retardation, autism and psychiatric disorders.
Given that there is also evidence for modifying loci (that is, the same MeCP2 mutations leading to discordant phenotypes), the full spectrum of phenotypes associated with MeCP2 mutations in both males and females has yet to be delineated exhaustively.
Fine tuning
The protein encoded by MeCP2 is a methyl-DNA binding protein believed to play a major role in gene regulation. Surprisingly, although MeCP2 mutations in mice lead to a phenotype remarkably similar to that seen in RTT females, they do not lead to gross differences in gene expression. Rather, MeCP2 seems to modulate or fine-tune the expression of genes whose on/off switch is determined in an MeCP2-independent manner.
A number of target genes whose expression is modulated by MeCP2 have been identified and include brain-derived neurotrophic factor (BDNF)4 and the neuropeptide corticosterone-releasing hormone (CRH)5, among others. BDNF expression is reduced in MeCP2-mutated mice, and BDNF conditional knockout mice display many phenotypes overlapping with MeCP2-mutated mice. Similarly, enhanced CRH expression in the MeCP2-mutated mice may explain a number of anxiety-related behaviors, suggesting CRH receptor antagonists as a potential therapeutic approach.
Although the best approach to developing therapeutic strategies for RTT remains unclear, recent work from the laboratories of Adrian Bird6 and Rudy Jaenisch7 hold substantial hope for the future. Working independently, both groups have shown that restoration of MeCP2 function in adult mice can reverse MeCP2-deficient phenotypes.
Despite the devastating phenotype associated with RTT, the neurons themselves appear not to be irreversibly damaged. In the ensuing decade since the description of MeCP2 as the gene responsible for RTT, a great deal has been learned and new research directions identified.
As has been the case with other autistic-like genetic disorders, such as fragile X syndrome, the discovery of the responsible gene serves as the basis from which further fundamental knowledge of the biochemistry, cell biology, and physiology of the disorder can be built, all in the hope of eventually developing effective interventions.
References:
- Amir R.E. et al. Nat. Genet. 23, 185-188 (1999) PubMed
- Hagberg, B. et al. Ann. Neurol. 14, 471-479 (1983) PubMed
- Amir R. et al. Am. J. Med. Genet. 90, 69-71 (2000) PubMed
- Chen W.G. et al. Science 302, 885-889 (2003) PubMed
- McGill B.E. et al. Proc. Natl. Acad. Sci. USA 103, 18267-18272 (2006) PubMed
- Guy J. et al. Science 315, 1143-1147 (2007) PubMed
- Giacometti E. et al. Proc. Natl. Acad. Sci. USA 104, 1931-1936 (2007) PubMed
Recommended reading
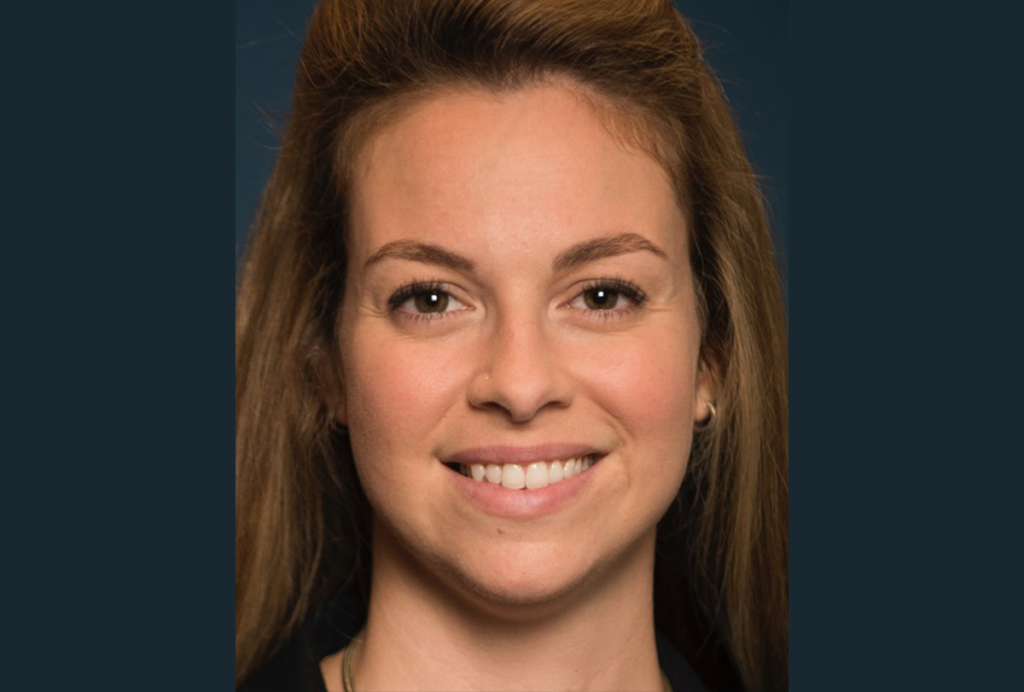
Autism program chief among National Institutes of Health layoffs
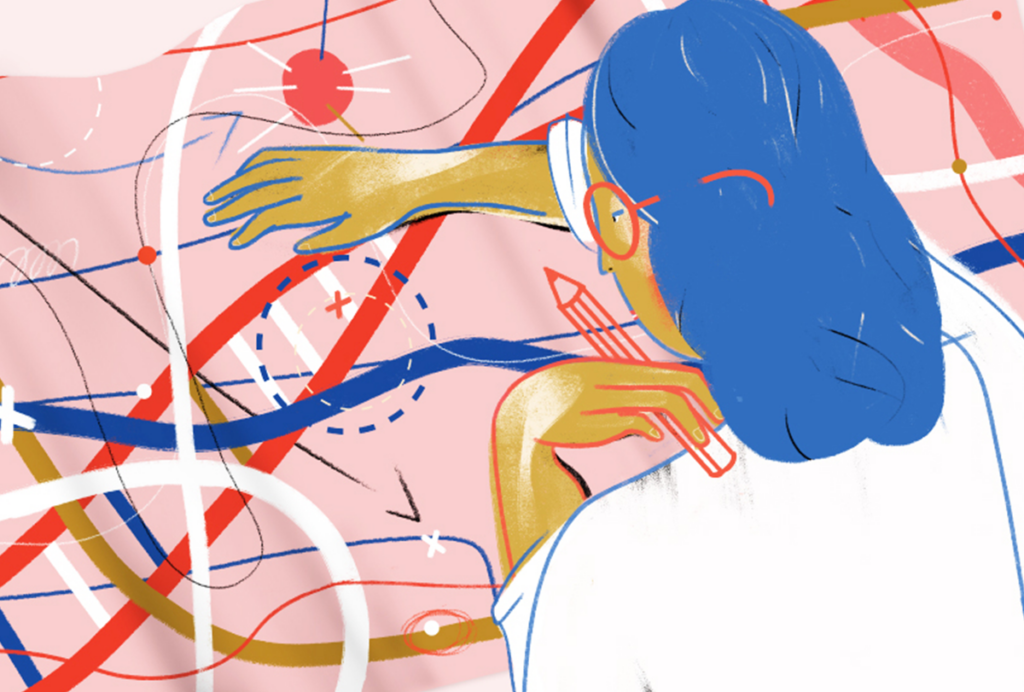
Familiar autism-linked genes emerge from first analysis of Latin American cohort
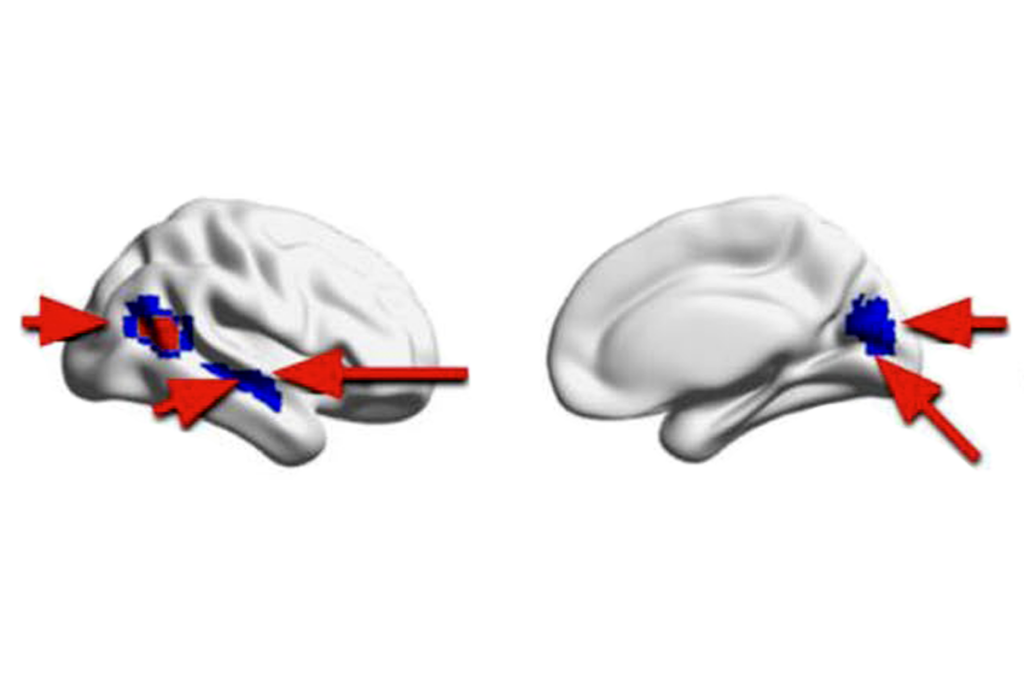
Depression perception; MYT1L mice; brain signal variability
Explore more from The Transmitter
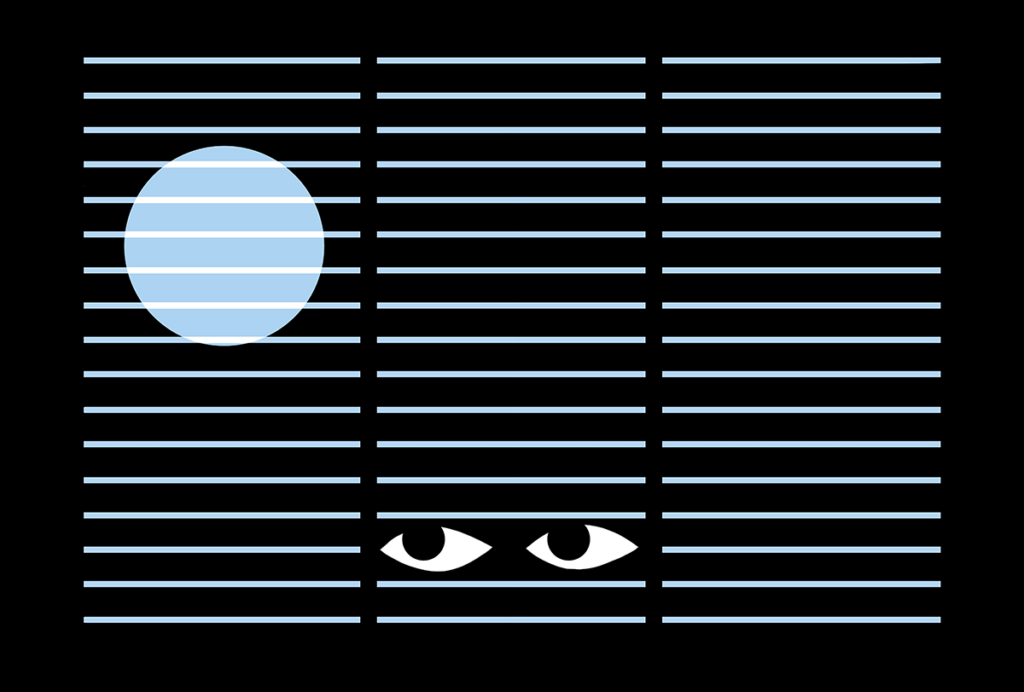
This paper changed my Life: Bill Newsome reflects on a quadrilogy of classic visual perception studies
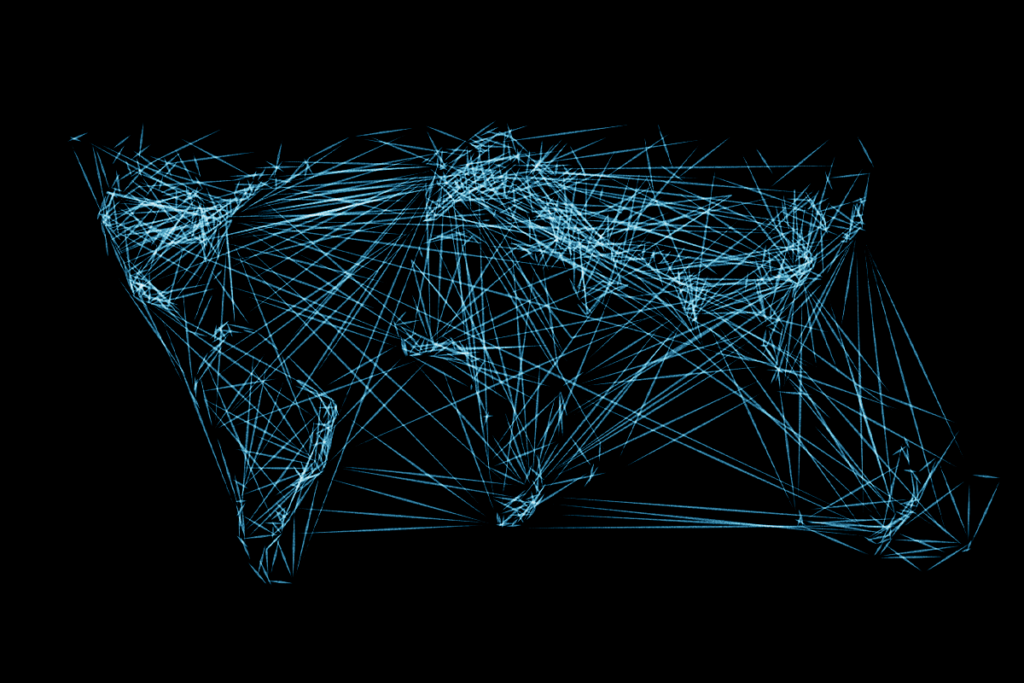
Science must step away from nationally managed infrastructure
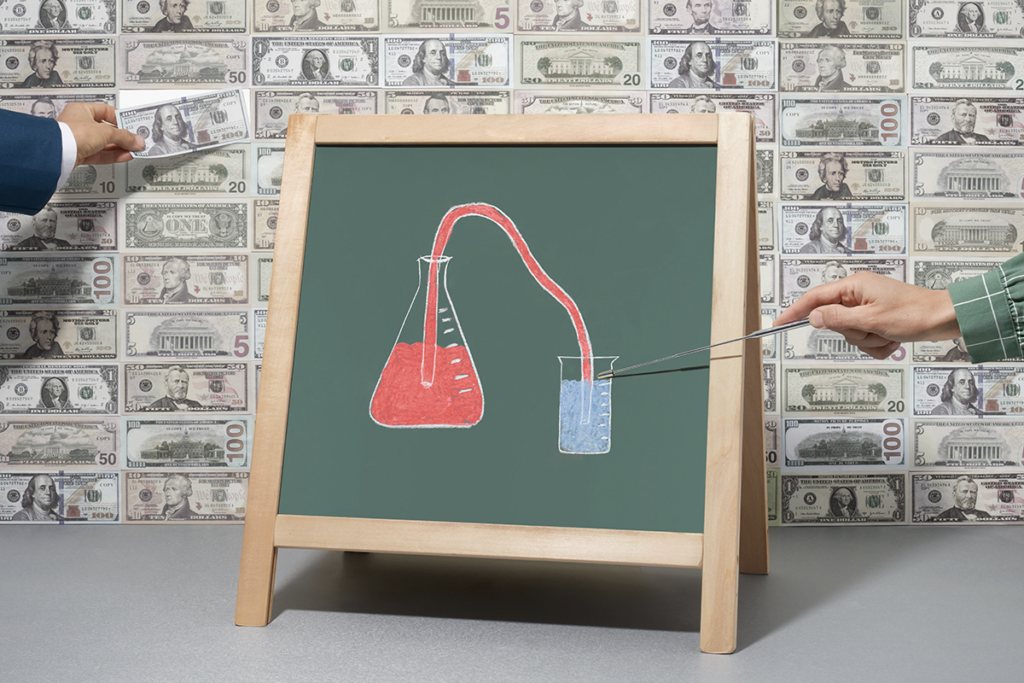