RNA binds to fragile X gene, shutting it down
Misplaced pieces of RNA bind and disable the gene responsible for fragile X syndrome, leading to the disorder, according to a study published 28 February in Science.
Misplaced pieces of RNA bind and disable the gene responsible for fragile X syndrome, leading to the disorder, according to a study published 28 February in Science1.
Researchers learned more than 20 years ago that a mutation that silences the FMR1 gene leads to fragile X syndrome, a disorder that causes intellectual disability and, often, autism. A mutated segment of the gene, composed of more than 200 repeats of a three-nucleotide (CGG) sequence, proved to be the culprit2. But the mechanism by which the CGG repeats shut off the FMR1 gene had remained a mystery until now.
It turns out that messenger RNA (mRNA), which acts as the template for proteins, can derail protein production as well. Copies of mRNA with the fragile X mutation bind to repeats in FMR1, shutting down the gene’s expression.
“What we found was that the mRNA can come back and turn off the gene in a completely new way,” says Samie Jaffrey, professor of pharmacology at Weill Cornell Medical College in New York, who led the study.
Other experts laud the study as an important step in solving a long-standing mystery.
“It’s exciting to me because 20 years beyond the identification of the mutation, we really didn’t have any clue how this happened,” says David Nelson, professor of molecular and human genetics at Baylor College of Medicine in Houston, who was not involved in the new research. “This is a nice advance.”
Cellular silencing:
Standard methods were unable to determine the specifics of the silencing mechanism. When the human FMR1 gene containing CGG repeats is inserted into a mouse or another animal model, for example, the gene never shuts off, making it impossible to study the process in those models.
“The gene only gets silenced in real human beings, at about 11 weeks of embryonic development, when it is turned off, and it stays off forever,” Jaffrey says.
Attempting to observe how and when FMR1 is silenced using human induced pluripotent stem cells — adult cells returned to an embryonic state — didn’t work either.
Although these reprogrammed cells had lost their adult identities, they retained the markers laid down during development that had turned off FMR1. Because the gene stayed silent, scientists could not study the shutoff mechanism.
Jaffrey and his colleagues instead turned to embryonic stem cells, in which the gene is still active. They coaxed cells from two human stem cell lines, each with well over 200 CGG repeats in FMR1, to differentiate into neurons. They then observed the cells’ development for 60 days.
For the first 48 days or so, the FMR1 gene in the cells functions normally, producing normal amounts of mRNA and FMRP. After that, however, the levels of both mRNA and the protein drop off quickly. By 51 days, both are absent from the cell, indicating that the FMR1 has been silenced.
To find out whether mRNA is involved in the silencing, the researchers used a virus to delete the mRNA from the stem cells. This averted the silencing of the gene, suggesting the mRNA plays a key role in the process.
The scientists then introduced a small molecule called 1a, which prevents the CGG repeats in the mRNA from binding to anything. The molecule prevents FMR1’s shutdown. “We found that if we applied the [small molecule] very early, we could prevent silencing from happening in the first place,” Jaffrey says.
“This raises the interesting possibility that this drug could be used as an in utero therapy for fetuses that seem to have the fragile X [mutation], to delay or prevent the gene silencing,” Jaffrey says.
Other experts say this approach is promising but note that the road to the clinic is not likely to be straightforward.
“With a small molecule, you always have to ask about the toxicity and how it could impact other gene expression,” says Peng Jin, professor of human genetics at Emory University in Atlanta, who was not involved in the study.
Still, knowing the mechanism may help scientists take further steps, such as pinpointing the changes at 11 weeks that make the silencing possible, notes Nelson. “Maybe we can then create a mouse model by changing those, which I think will in some ways be easier to study.”
References:
1: Colak D. et al. Science Epub ahead of print (2014) PubMed
2: Verkerk A.J. et al. Cell 65, 905-914 (1991) PubMed
Recommended reading
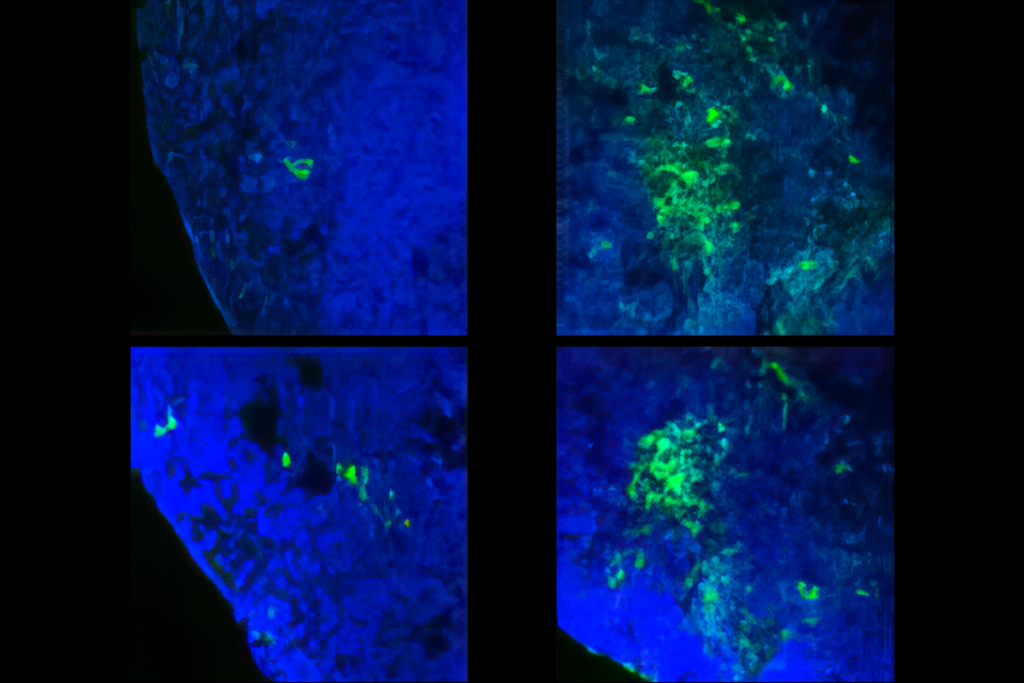
Personalized medicine; astroglia organoids; fast track for fragile X drug
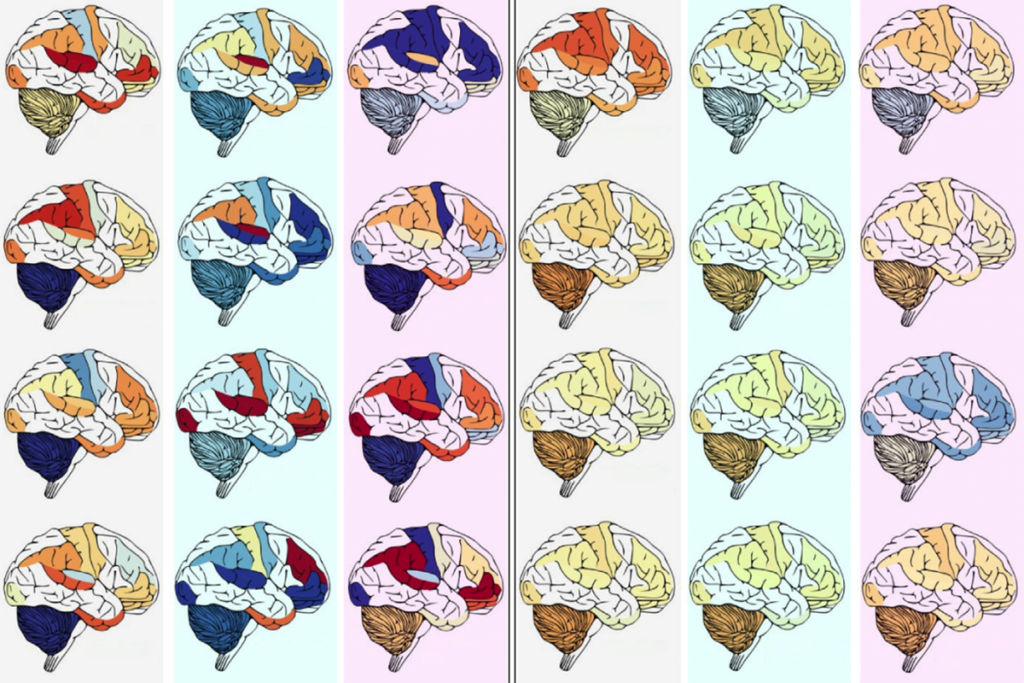
X marks the spot in search for autism variants
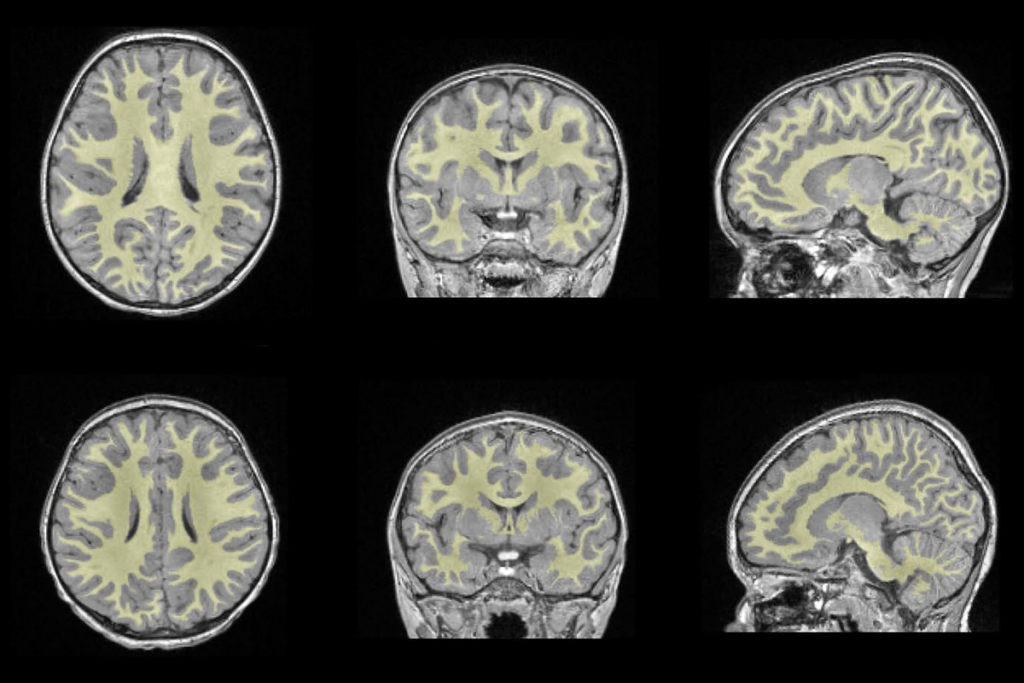
White-matter changes; lipids and neuronal migration; dementia
Explore more from The Transmitter
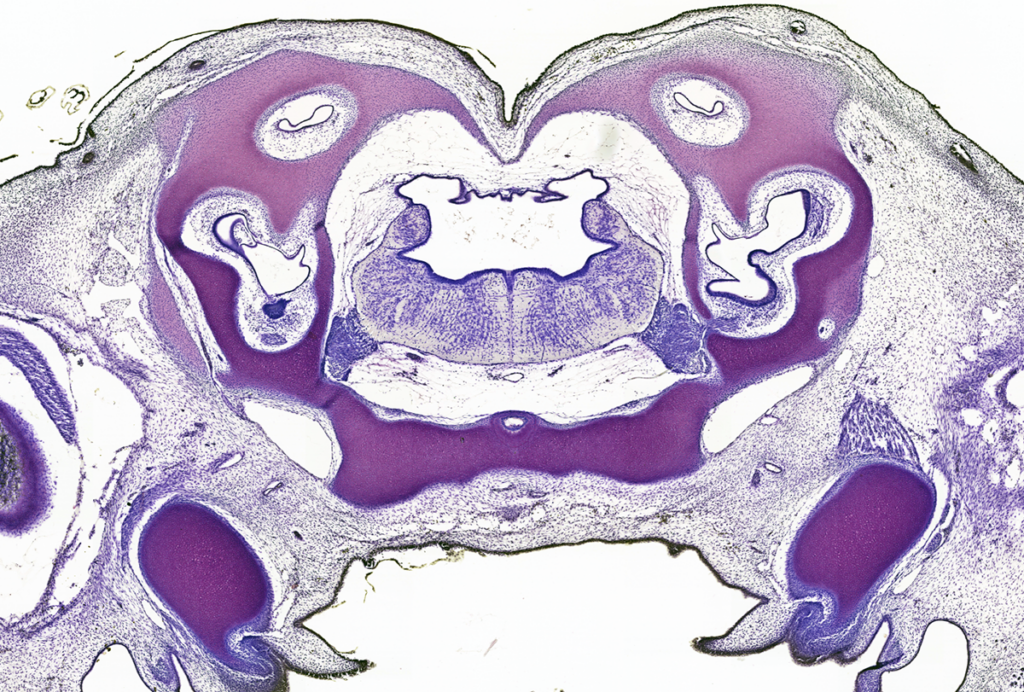
Digitization of ‘breathtaking’ neuroanatomy slide collection offers untapped research gold mine
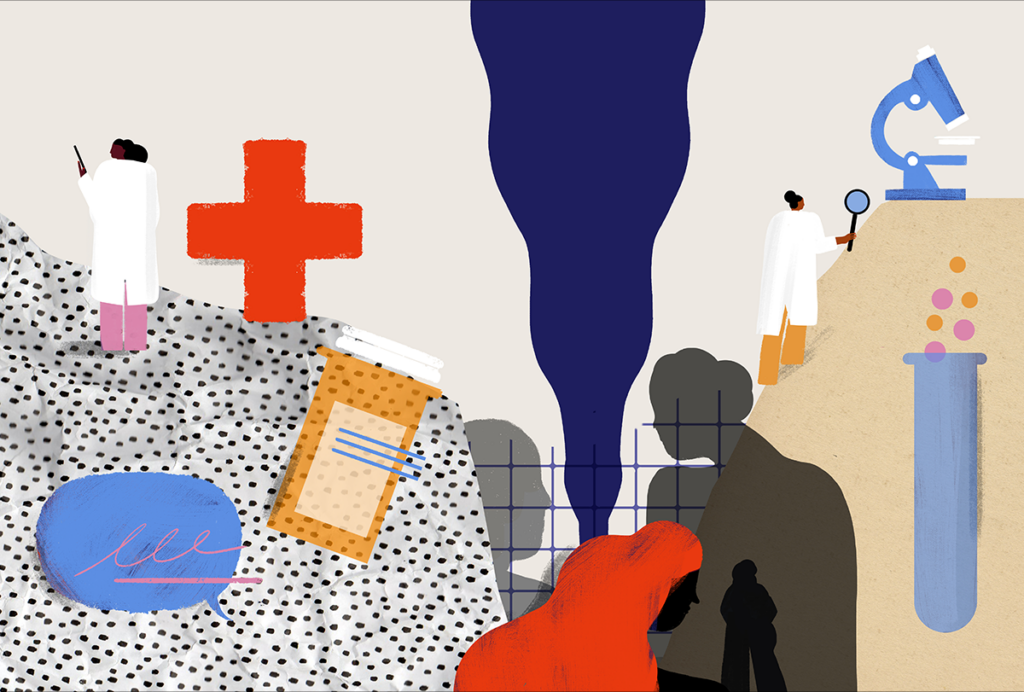
Neuroscientists need to do better at explaining basic mental health research
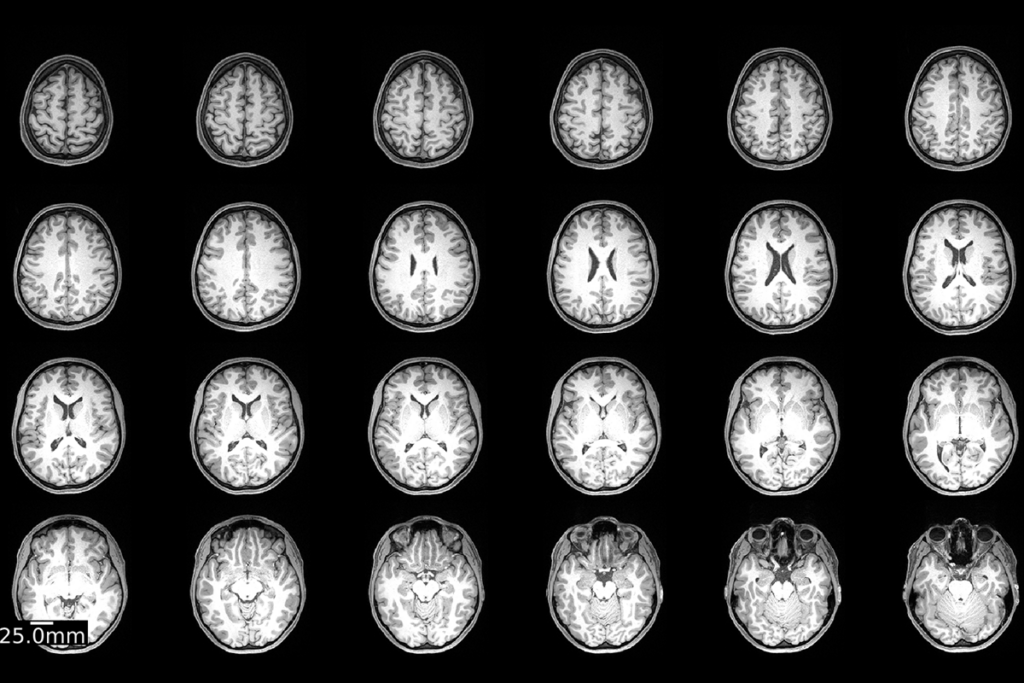