Questions for Timothy Murphy: Mice take selfies of their brains
By coaching mice to position themselves under a microscope, researchers can regularly peer into their brains without disrupting the rodents’ social life.
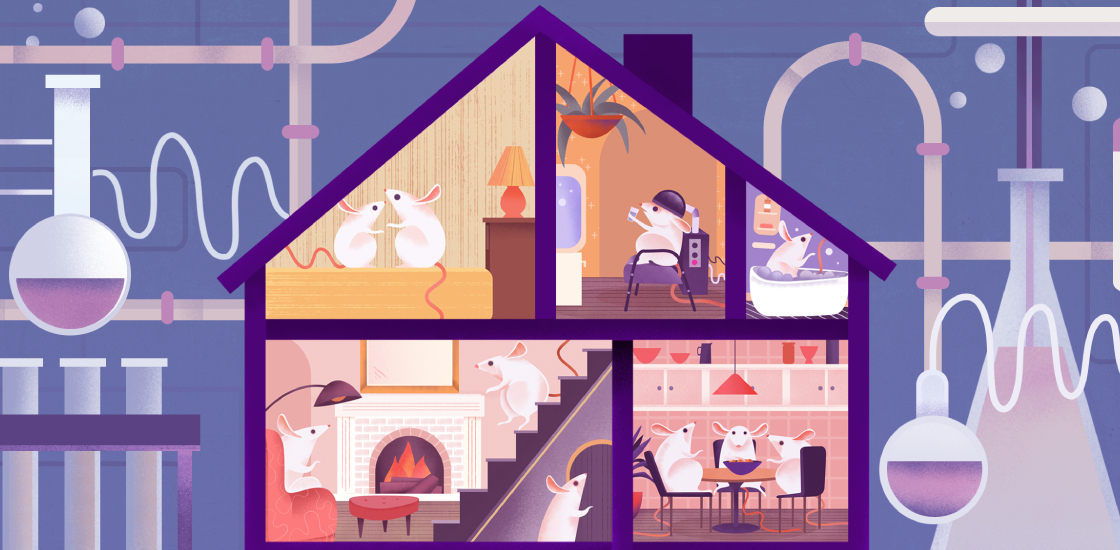
A laboratory mouse has a modest home: a small, smelly cage lined with soft bedding, which it shares with up to four other animals. But it is home nonetheless — a place of comfort. That is, until the massive hand of a researcher reaches in to pluck it out for an experiment.
The experiment might gauge whether a mouse feels anxious or social, or tap the activity in its brain. But does the intrusion of the researcher’s hand influence the very behavior under study?
Yes, says Timothy Murphy, professor of cellular and physiological sciences at the University of British Columbia in Vancouver, Canada. Murphy’s team has developed a high-tech cage that allows a mouse to go about its business uninterrupted1. The cage records the mouse’s every move. Whenever the animal is thirsty, it enters a corridor, attaches its head to an apparatus, and takes a drink while a microscope takes a picture of its brain activity.
Murphy and his colleagues have used the cages to measure synchrony between mouse brain regions. In one experiment, the researchers captured more than 7,000 snapshots of brain activity in less than two months — all of them after the mice voluntarily ‘posed’ for the camera.
We asked Murphy how he trains mice to participate, and how this approach could help autism research.
Spectrum: How does your imaging approach work?
Timothy Murphy: We need to prep each mouse first. For this type of imaging, we part the skin on their heads and glue a piece of glass over the skull that provides a flat surface for imaging. But unlike other methods, we do not need to make a window into their skulls. If we are imaging interactions between brain regions (and not single neurons) we have sufficient resolution through the intact mouse skull.
We attach a bar to each mouse’s head that helps lock the animal into position under a microscope. We then tag each mouse with a small device, the size of a grain of rice, that emits radio frequencies, which we detect with sensors on a computer outside the cage. This allows us to track and identify each mouse.
We then train the mice to attach themselves to a microscope at the end of a tunnel attached to the cage. Before the training starts, we restrict their water so they’re motivated to find water. The water source is through the tunnel, and the only way the mice can fit into this tunnel is if they enter it in a way that places the bar on their head onto a track. As soon as they start down the track, computer software detects the mouse’s radio frequency and sends a signal to a waterspout to release water. Over time, we move the waterspout farther from the entry point so that the mouse has to go deeper into the corridor.
When a mouse gets to the end of the corridor, the bar contacts sensors. These sensors trigger a mechanical system that pins the bar in place under the microscope and immobilizes the mice for up to two minutes as they drink.
Once the mice realize this is the path to getting a lot of water, they are on board. They come and go several times a day.
S: What is the advantage of this setup?
TM: The traditional approach requires that researchers remove the mice from their home cage in the animal facility and transport them to the lab where they do the experiments. Researchers usually restrict the animals’ water and food so that they get it all at once during the test, to motivate the mice to complete the testing. Physiologically, this may be damaging for the animal.
In our experiment, we give the mice a choice. They need to enter the chamber to get water, but they can get as much as they want, whenever they want.
The mice also seem anxious when moved. Their stinky, soiled cage is home. And each cage has a social structure that you disrupt as soon as you pick up some of the mice and remove them. We’ve noticed that whenever we’ve had to move a mouse to another room, their participation in experimental tasks really changes. And the remaining mice may even notice when a mouse is removed. In one case, we had to take an animal because it had a problem with its head-mounted bar, and the other mice didn’t enter the corridor and drink as much after that — they seemed to miss the mouse.
Finally, the automated setup makes it easier to study nocturnal animals. A good mouse behavior group will keep its facilities dark during the daytime so that experiments occur when mice are naturally awake and active. But you still need to take the mice out of the cage and bring them into the lab or testing room, which may be difficult to keep dark. Researchers could use dim red lights or night vision goggles to handle the animals so they can stay in darkness, but that just gets really complicated.
S: Is this method useful for autism research?
TM: Yes. Our system allows us to keep the mice together and scan their brains without disrupting the social order. Each cage holds up to 5 mice, but we are now linking two cages together, to allow us to keep up to 10 mice together.
Because each mouse is tagged with a tracking device, we can house an animal carrying an autism-linked mutation along with control animals and compare their brain activity and social dynamics. To monitor social activity, we can use radio tags to map the location of each mouse and measure how much the mice interact. We also use streaming video, much like a nanny camera, to record and monitor the behavior of the mice 24 hours a day.
We can genetically modify the mice so we can activate certain neurons in their brains with beams of light — a technique called optogenetics. One theory of autism is that there is an imbalance between neurons that inhibit brain activity and those that excite it. So one possible experiment would be to alter this balance whenever certain mice went to drink to see whether that manipulation changes how these mice interact with their peers and move about the cage.
S: What do you plan to do next?
TM: Most of our efforts are aimed at optimizing this system. We still need to weigh the mice to make sure they are actually drinking the water and not getting dehydrated. So we’d like to develop a way to automatically weigh each mouse. That way, we can really keep those cages undisturbed for extended periods of time.
We also want to get the system in the hands of other investigators. We’re using inexpensive components. The cage is a few hundred dollars to build and it uses a $35 computer that is the size of a credit card. Our website provides the code for the software that detects individual mice and controls the microscope, as well as a three-dimensional printing file to make the head-fixing tube. In these ways, we’re working to put forward the best and most accessible platform for the research community.
References:
- Murphy T.H. et al. Nat. Commun. 7, 11611 (2016) PubMed
Syndication
This article was republished in Scientific American.
Recommended reading
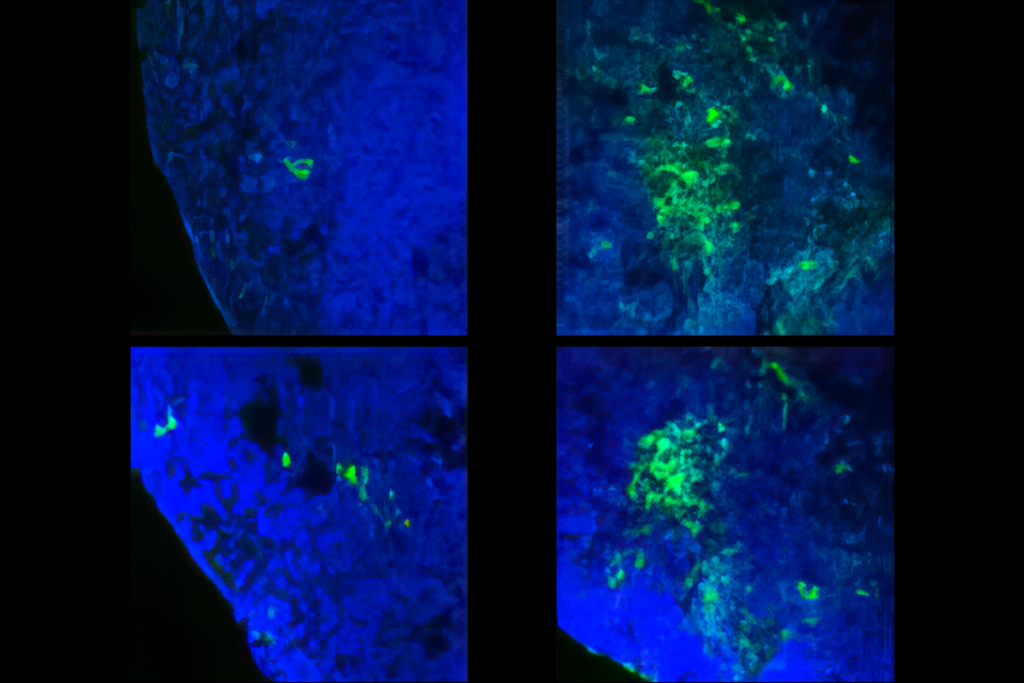
Personalized medicine; astroglia organoids; fast track for fragile X drug
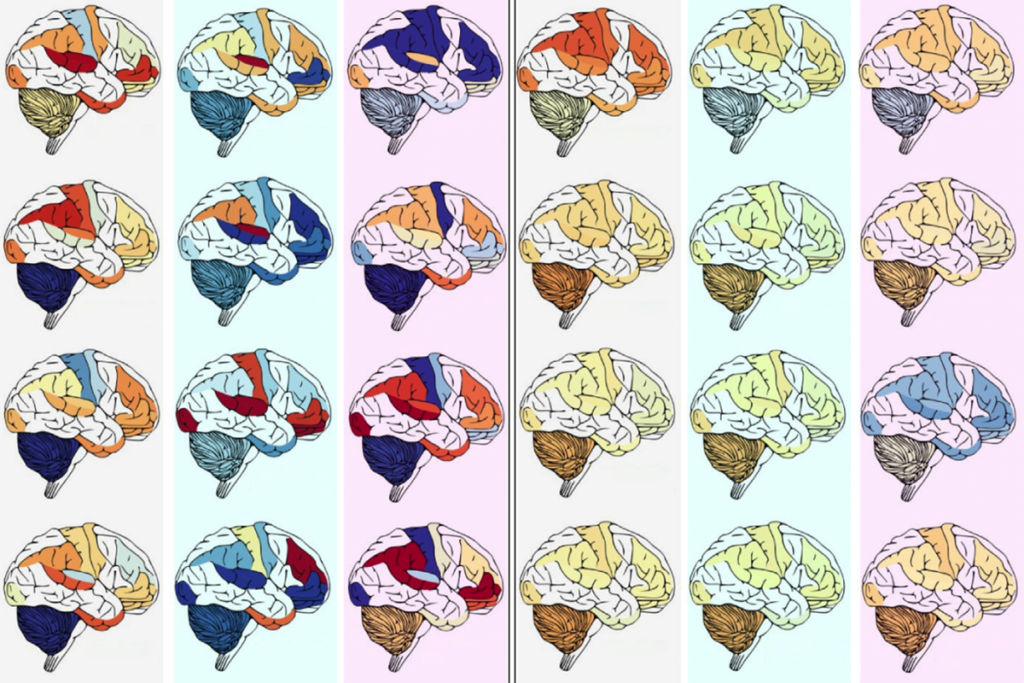
X marks the spot in search for autism variants
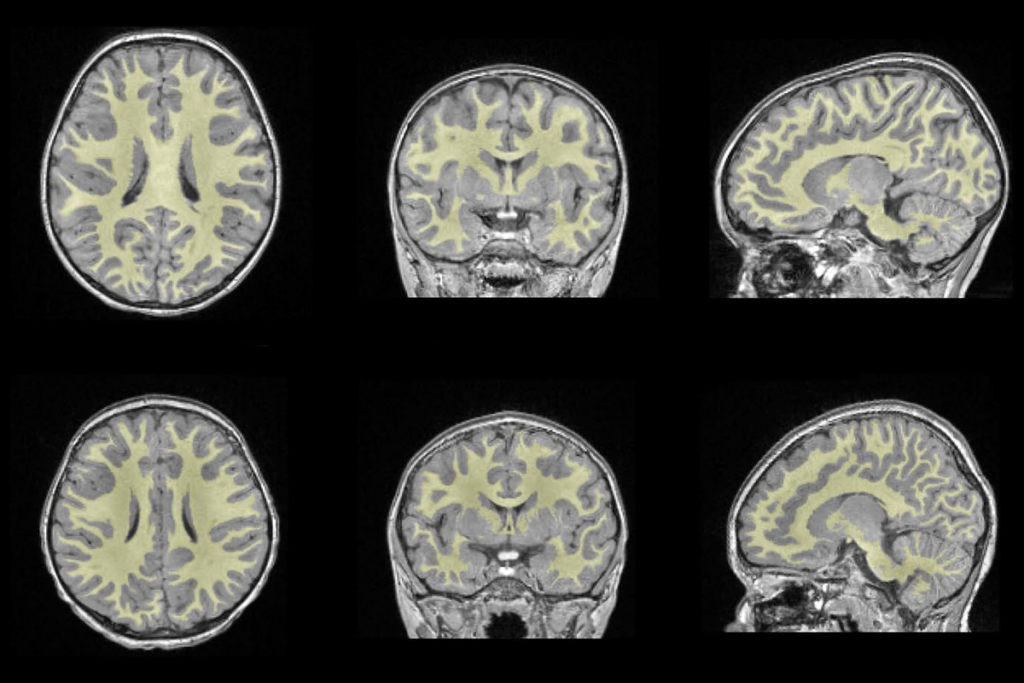
White-matter changes; lipids and neuronal migration; dementia
Explore more from The Transmitter
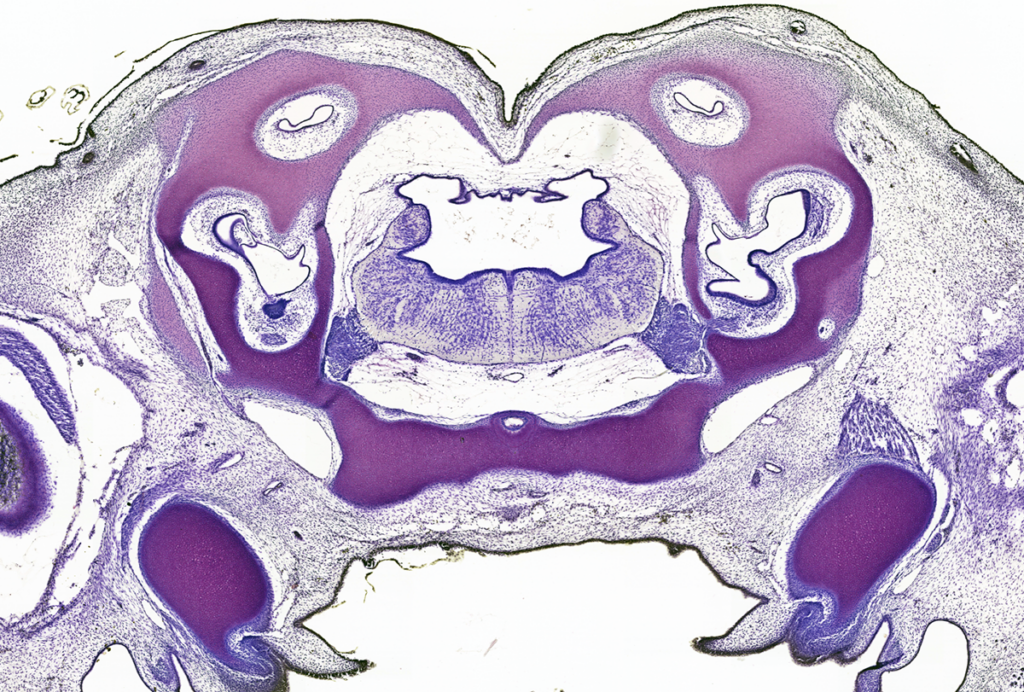
Digitization of ‘breathtaking’ neuroanatomy slide collection offers untapped research gold mine
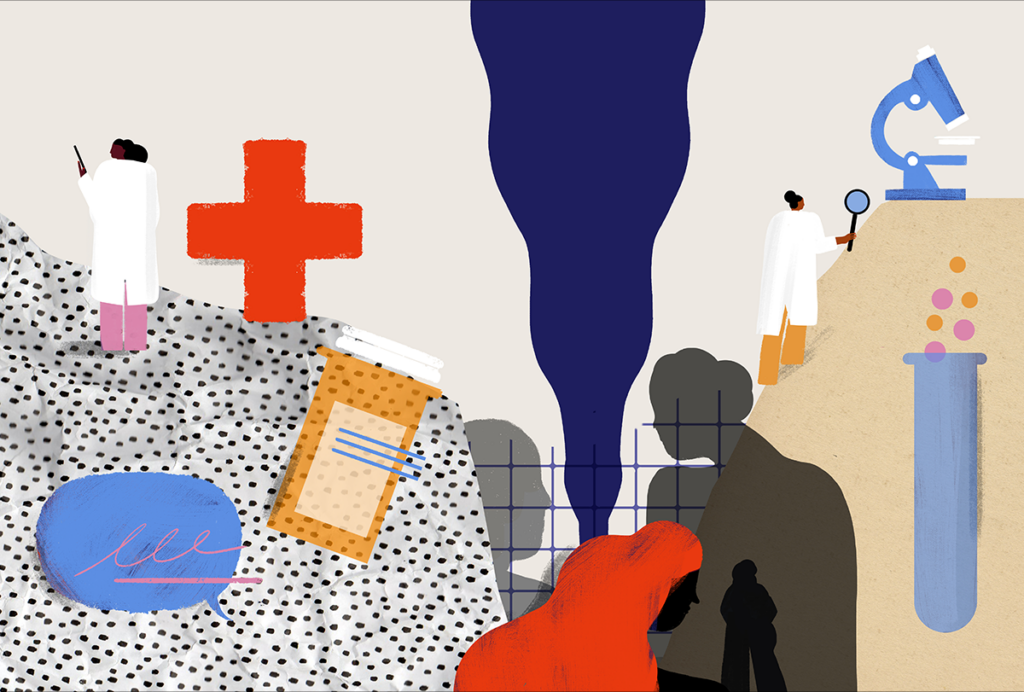
Neuroscientists need to do better at explaining basic mental health research
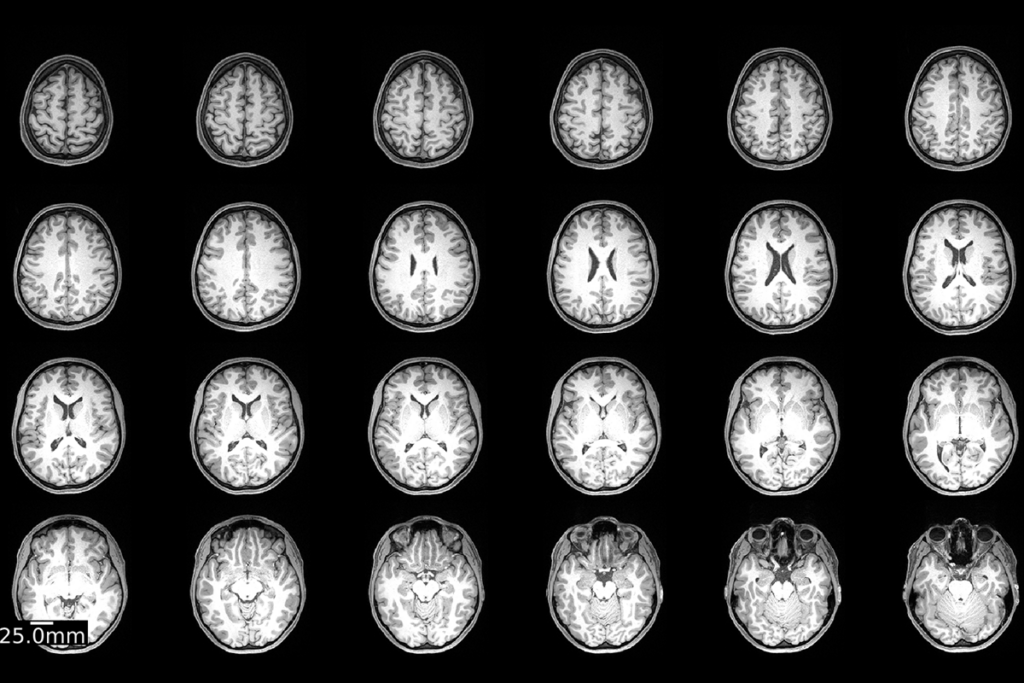