Brain’s bridge could yield clues to faulty wiring in autism
Decoding distortions in the brain’s largest nerve tract could lay bare basic problems with long-range neural connections in autism.
Autism stems from problems with connectivity, or communication between distant parts of the brain. As autism theories go, that one has solid support. For that reason, the corpus callosum deserves special attention.
The corpus callosum is, in essence, an information superhighway that carries a large volume of neural traffic across the brain. It is a thick bundle of nerve fibers that bridges the right and left halves of the brain. It is also the brain’s largest tract of white matter, the neuronal fibers that connect different brain regions.
“The corpus callosum is the quintessential example of how important the white matter is for brain region communication,” says Thomas Frazier, director of the Center for Autism at Cleveland Clinic Children’s in Ohio.
There are numerous studies linking the corpus callosum to autism. Yet every time scientists draw a conclusion about its role in the condition, evidence shows up to contradict it.
Since the 1990s, for example, many studies have revealed that people with autism tend to have a smaller corpus callosum than controls do. In a study published in January, Italian researchers found that preschoolers with autism who have a small corpus callosum also have more severe autism features than others on the spectrum1. Imaging studies have also consistently shown that the corpus callosum has poorer structural integrity in school-age children and adults with autism than it does in controls2.
However, other studies have revealed that some people with autism have an enlarged corpus callosum. And still other data show that alterations in its size and structure in people with autism may evolve and even reverse direction with age.
Taken together, these results suggest that understanding the corpus callosum’s role in autism will require studying the structure at multiple ages. In the process, scientists may learn how changes in white matter — in the corpus callosum and throughout the brain — relate to autism traits.
Broken bridge:
About 1 in 4,000 people are born lacking all or part of the corpus callosum. These individuals have difficulties with social interaction that are reminiscent of those seen in autism. The latest data suggest that about one-third of people with the brain abnormality known as agenesis of the corpus callosum, or AgCC, meet the diagnostic criteria for autism.
Even people with AgCC who don’t have autism often have problems with social skills. The corpus callosum’s connective power supports complex social interactions, says Lynn Paul, senior research scientist at the California Institute of Technology. People with AgCC can’t keep up with everyday social interactions, she says. “And as soon as you start missing information, you start misinterpreting things, and it snowballs.”
There are genetic links between AgCC and autism as well. The first large study to look at genetic overlap between the two conditions found that large deletions or duplications of DNA affect similar regions of the genome in both. A few genes have also been implicated in both conditions. These include ANK2 and EPHB, which help direct neurons to their proper places during development.
The findings support the idea that the social difficulties in autism may arise from a weak bridge between the brain’s hemispheres, as they do in people with AgCC.
However, the story is unlikely to be so simple: Emerging evidence links an enlarged corpus callosum to autism, too.
For example, individuals with autism who have mutations in a gene called PTEN have markedly enlarged heads, or macrocephaly. This unusual trait may result from changes in the brain’s white matter, especially the corpus callosum, according to a 2014 study. “[The participants’] corpus callosum was huge,” says Frazier, who led the study.
People who carry a deletion of chromosomal region 16p11.2, which is linked to autism, also have enlarged heads; those with a duplication of the region have unusually small heads. Unpublished data suggest that the changes in brain size preferentially affect the size of the corpus callosum.
“Even after correcting for the fact that you expect the brain to be larger or smaller, the corpus callosum was disproportionately bigger or smaller still,” says Elliott Sherr, professor of neurology at the University of California, San Francisco, who led that work.
How an enlarged corpus callosum might lead to autism is unclear. One possibility is that an excess of nerve fibers in the bridge creates complications. “If efficiency is the goal, you can have efficiency being diminished by the absence of connections, but you can also have efficiency being interrupted by too many connections,” Sherr says.
Loose connections:
Mouse models of PTEN mutations suggest a different possibility. In these animals, the fatty substance known as myelin that wraps around and insulates long nerve fibers appears loose and “unraveled,” Frazier says3.
This suggests that abnormally developed white matter, rather than too much or too little of it, drives autism features, Frazier says.
An even more nuanced picture emerges from studies that track people with autism over time. The studies have found that the corpus callosum changes all through development and into adulthood — both in its overall size and the structure of the fibers that traverse it.
In January, Eric Courchesne’s team at the University of California, San Diego reported that before age 2, children who will later be diagnosed with autism show evidence of an abundance of unusually small nerve fibers in the corpus callosum4. But these signs disappear by age 4, consistent with slowed development of neurons in children with autism.
Jason Wolff’s team at the University of Minnesota followed 270 siblings of children with autism from the age of 6 months to 24 months. These ‘baby sibs’ are at increased risk of autism. The researchers found that baby sibs who would later be diagnosed with autism had a thicker corpus callosum at 6 to 12 months old than either siblings without autism or controls. But by 24 months, those differences had almost disappeared.
Another effort involved 100 boys and men with autism aged 3 to 35 years. Researchers scanned their brains multiple times over nine years, and found that the corpus callosum is abnormally large in early childhood, but shrinks relative to controls after age 10.
The latter two studies also indicate that white matter fibers in the corpus callosum of people with autism are unusually robust early in life, but weaken significantly over time5. Although the age ranges of these two studies don’t overlap, “[when] you put the graphs up next to each other, you get a sense that the pieces of the puzzle might fit,” says Brittany Travers, assistant professor of kinesiology at the University of Wisconsin-Madison, who worked on the second study.
As a result of this body of work, researchers are focusing on whether the corpus callosum develops atypically in autism, rather than whether it is abnormal at a particular point in time, says Jed Elison, assistant professor of child development at the University of Minnesota. The longitudinal findings “really changed the way we think about the role of the corpus callosum in autism,” he says.
In Courchesne’s study, the discrepancies are most apparent in nerve fibers that connect the temporal lobes of the brain. This finding suggests that researchers should map subsets of fibers in the corpus callosum, rather than looking at the structure as a whole.
Social ties:
Meanwhile, how unusual development of the corpus callosum relates to autism’s features remains a mystery. In the baby sibs study, Wolff and his team found that the infants with the largest corpus callosum also show the most repetitive behaviors at age 2. But Travers and her team found no such connection between structure and behavior.
To explore this relationship, Paul and Elison are aiming to compare the early social development of children with AgCC to that of children with autism. So far, they have pilot data from about 70 families worldwide. Parents have completed extensive online questionnaires about their children at various ages between 6 months and 3 years.
The researchers are seeking funding to expand the study and compare their data with behavioral information from an ongoing study of baby sibs. Differences between the two groups might provide clues about the role of the corpus callosum in social cognition, Elison says.
Along similar lines, Frazier is conducting a long-term study to find the interconnections between autism features, PTEN levels and brain structure. Studying people with PTEN mutations or syndromes such as AgCC could be key to understanding the role of white matter and the connections between distant brain regions.
References:
Recommended reading
Assembloids illuminate circuit-level changes linked to autism, neurodevelopment
Explore more from The Transmitter
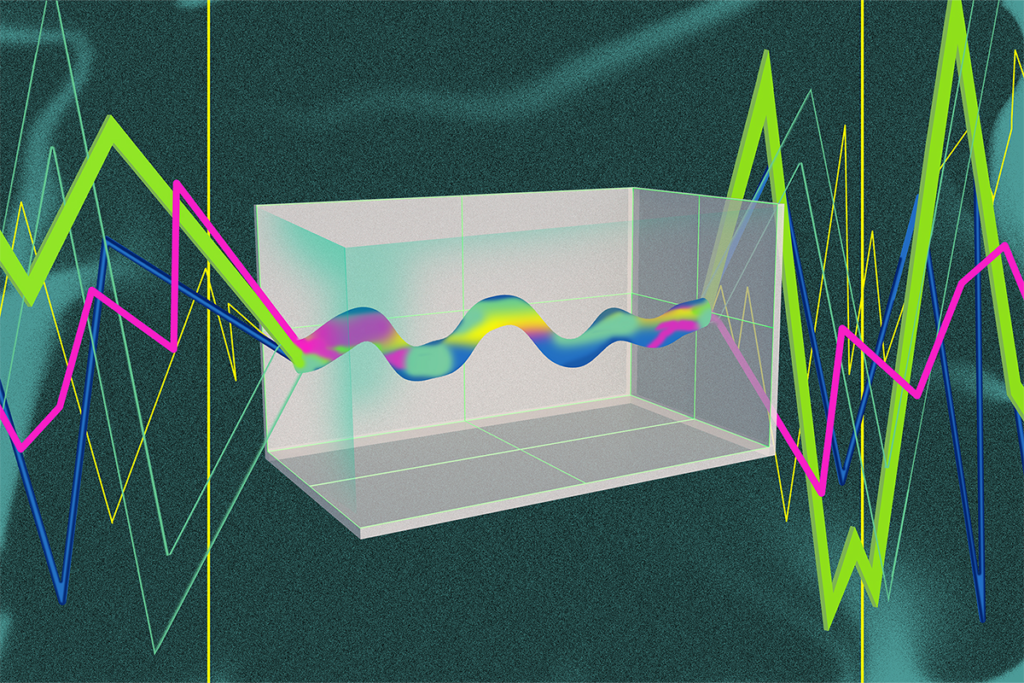
The Transmitter’s favorite essays and columns of 2024
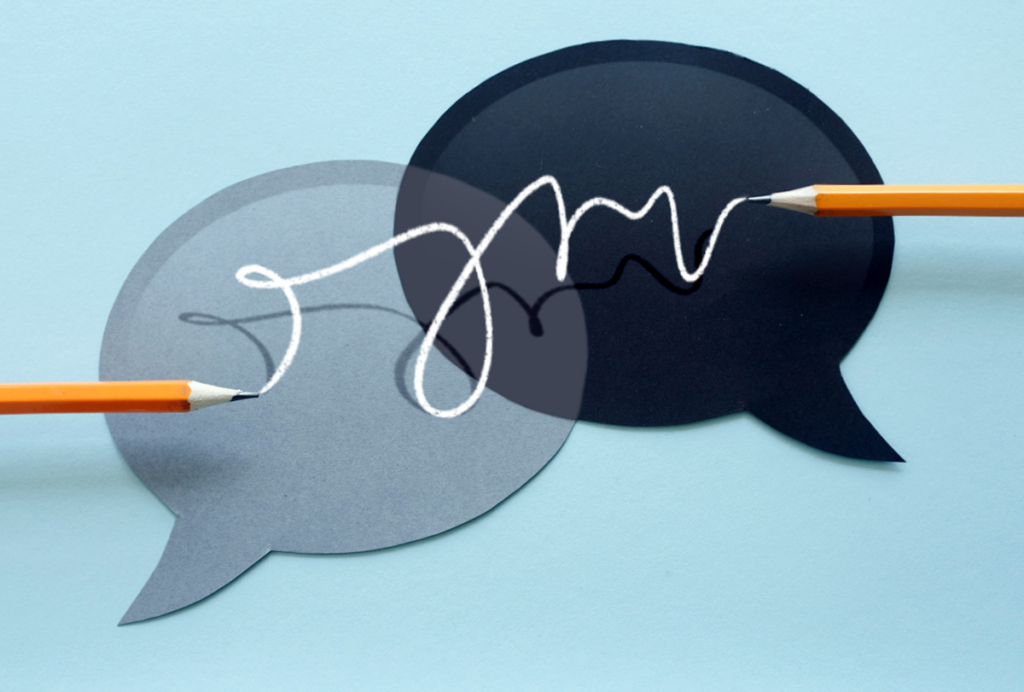
Say what? The Transmitter’s top quotes of 2024
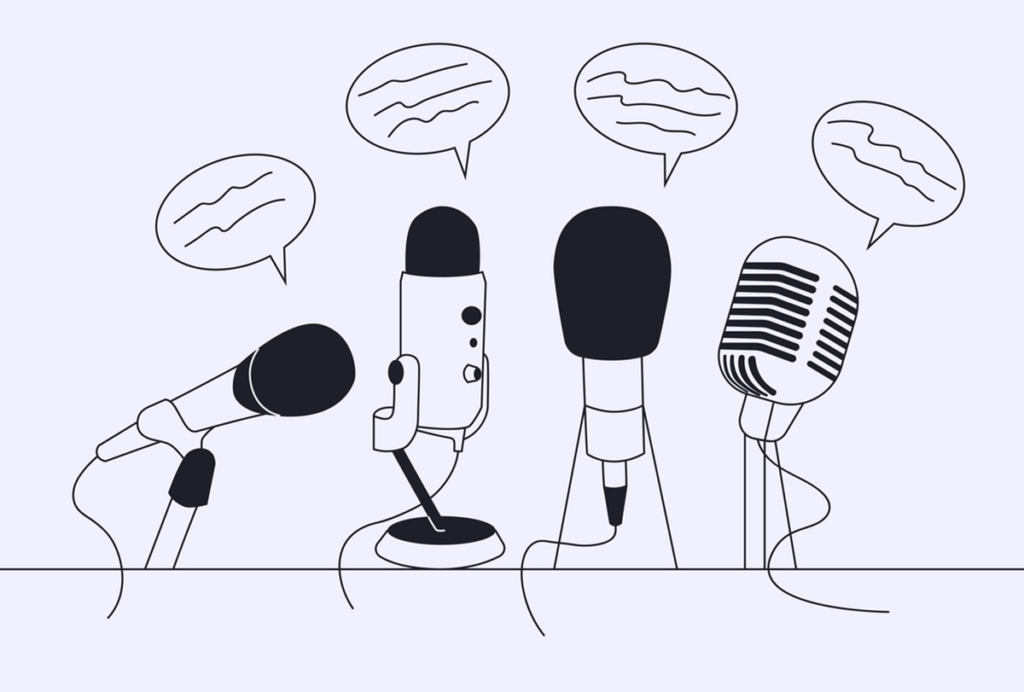