Brain imaging reveals simple organization for neural wires
Rather than a tangled bowl of spaghetti, the neural wiring in the brain is arranged in an orderly fashion, like a woven piece of cloth, according to research published today in the journal Science.
Rather than a tangled bowl of spaghetti, the neural wiring in the brain is arranged in an orderly fashion, like a woven piece of cloth, according to research published today in the journal Science1.
“While the brain looks like a very complicated thing, it has a basic three-dimensional grid, like the hallways and elevators we use to navigate a skyscraper,” says Paul Thompson, professor of neurology at the University of California, Los Angeles, who was not involved in the research.
The striking findings, which emerged from a combination of advanced brain imaging and analysis techniques, present an elegant geometry of the brain that researchers say could help explain how this complex organ develops and how it evolved.
“There are clear implications for understanding how the brain develops,” says Tim Behrens, university research lecturer at the University of Oxford in the U.K., who was not involved in the study. Rather than trying to understand the massive puzzle of a bowl of spaghetti, “if we know the rules for how the spaghetti is organized, we can work out how it [develops] from start to finish.”
A better understanding of brain development could in turn provide a new framework for studying what goes wrong in neurodevelopmental disorders, including autism.
In the new study, Van Wedeen, assistant professor of radiology at Massachusetts General Hospital, and his collaborators analyzed the cerebral fibers in humans and in four nonhuman primate species using a variation of magnetic resonance imaging called diffusion spectrum imaging. This technology detects the flow of water along the brain’s white matter fibers — the long wires that connect neurons — and uses that information to calculate their direction.
Lonely pathways:
Past efforts to map the brain’s white matter have provided only a limited road map. The traditional method, which uses molecular markers to directly trace fibers in the brain, can only be performed in animals, and can typically resolve a small portion of fibers at a time.
And unlike Wedeen’s method, which he has been developing and refining for the last ten years, most diffusion brain imaging approaches cannot resolve the areas where fibers intersect, again making it difficult to generate a complete picture of their organization.
“Not a lot of people have access to this kind of data,” says Behrens. “[Wedeen’s team] has the world’s leading technology for seeing these things.”
In the new study, the researchers painstakingly imaged the brains of humans and nonhuman primates. In the latter case, they used postmortem brains, running scans that sometimes lasted more than 24 hours.
The resulting maps are “astonishingly simple,” says Wedeen. “The fibers invariably form two-dimensional sheets, with a laminate structure like pages in a book.” Furthermore, when you look at a point where the sheets cross, “it always contains fibers that looked like woven fabric, with a weft and warp,” he says.
The stacks of fiber sheets are then folded as a single slab, “like a highly curved crystal,” says Wedeen. And when they change direction, the fibers appear to curve only at 90-degree angles.
Previous research had hinted at a geometrical organization in certain parts of the brain, such as the brainstem, and the motor and sensory parts of the cortex, which have a topographical layout. But Wedeen’s work is the first to show that this appears to be a fundamental organizing principle of the cortex.
One of the strengths of the paper is that the researchers confirmed their findings by doing traditional fiber tracing in primate brains.
That’s an unusual bonus, says Olaf Sporns, professor of psychology and cognitive neuroscience at Indiana University in Bloomington, who was not involved in the research. “The use of nonhuman primates and postmortem brains is not widely done because it requires a lot of expertise to do postmortem imaging really well.”
Sporns, who studies the brain’s functional networks, says the findings “remind us that the brain is not only a topographical network but also a network in space. The geometric relationships of fiber pathways and how they relate in three-dimensional space matters in development.”
New development:
The new work is likely to spark a flurry of research.
“Suddenly we have a gold mine of information,” says Thompson. “Even if you don’t use the imaging method [Wedeen] developed, you can use this principle to analyze your own data.”
Oxford’s Behrens says he saw evidence for these sheets in his own data several months ago and at first didn’t know what to make of it. “I think [Wedeen] has been able to convincingly show that the sheet structure is real,” says Behrens. But he is less certain about fiber tracts being limited to turns of 90-degree angles, which will need to be confirmed using other types of diffusion imaging.
Researchers say it will also be interesting to combine the new findings with one of the hottest areas of brain imaging research in recent years: the analysis of functional networks and how information moves along them.
“What I would find intriguing in the future is to try to understand how the geometric principle affects brain function, the actual flow of information in the brain network,” says Sporns.
Though what this new geometry means for brain function is not yet known, the researchers say it has clear implications for understanding how the brain develops.
Previous studies of embryonic development have shown that there are chemical gradients running from head to tail, front to back and left to right that guide cell growth along these gradients.
“So it stands to reason that this [grid] structure is a remnant of a simpler structure,” says Wedeen.
In the case of white matter fibers, Wedeen hypothesizes that gradients of three different chemicals could direct axons, or nerve fibers, to grow in three-dimensional space along the x-, y- and z-axis.
“That would give a very simple basis to build what is eventually a very complex latticework,” says Thompson. And once scientists begin to understand the normal development of the latticework, they can more easily “discover what kinds of things go wrong in the group of brain disorders thought to be related to wiring, including autism and schizophrenia,” he says.
Wedeen’s study is part of the Human Connectome Project, a $40-million effort funded by the National Institutes of Health to map the brain’s circuits and understand how these circuits govern function and behavior. As part of the project, the researchers have developed an even higher resolution scanning technology that will allow them to analyze the human brain in greater detail.
“It’s like the difference between looking at hill in the Catskills and an arch in the Painted Desert,” Wedeen says.
References:
- Wedeen V.J. et al. Science 335, 1628-1634 (2012) Abstract
Recommended reading
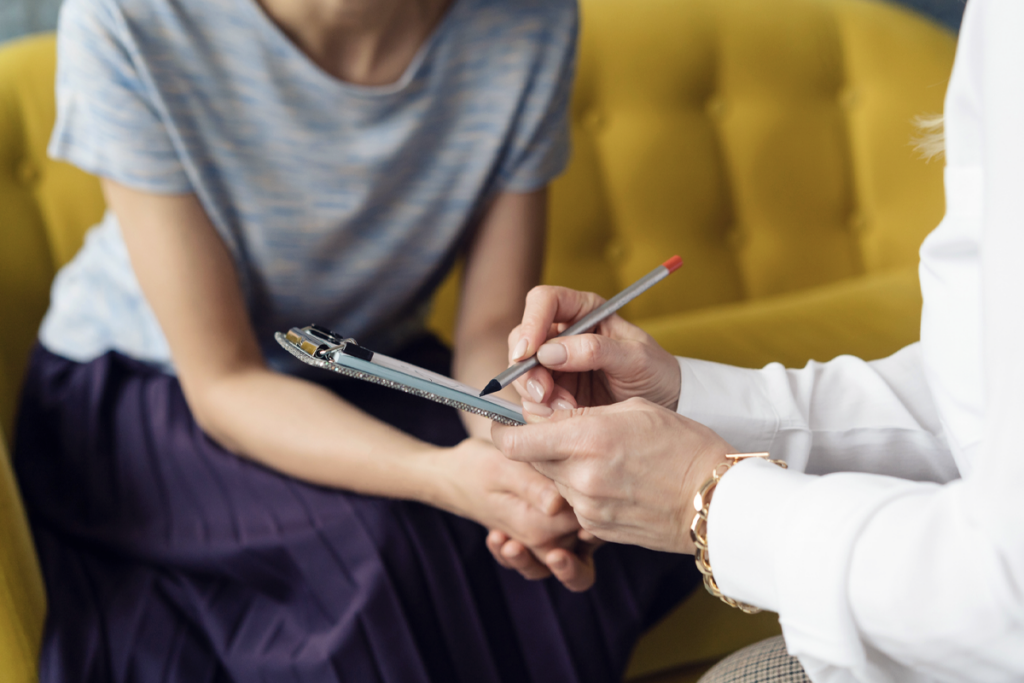
Expediting clinical trials for profound autism: Q&A with Matthew State
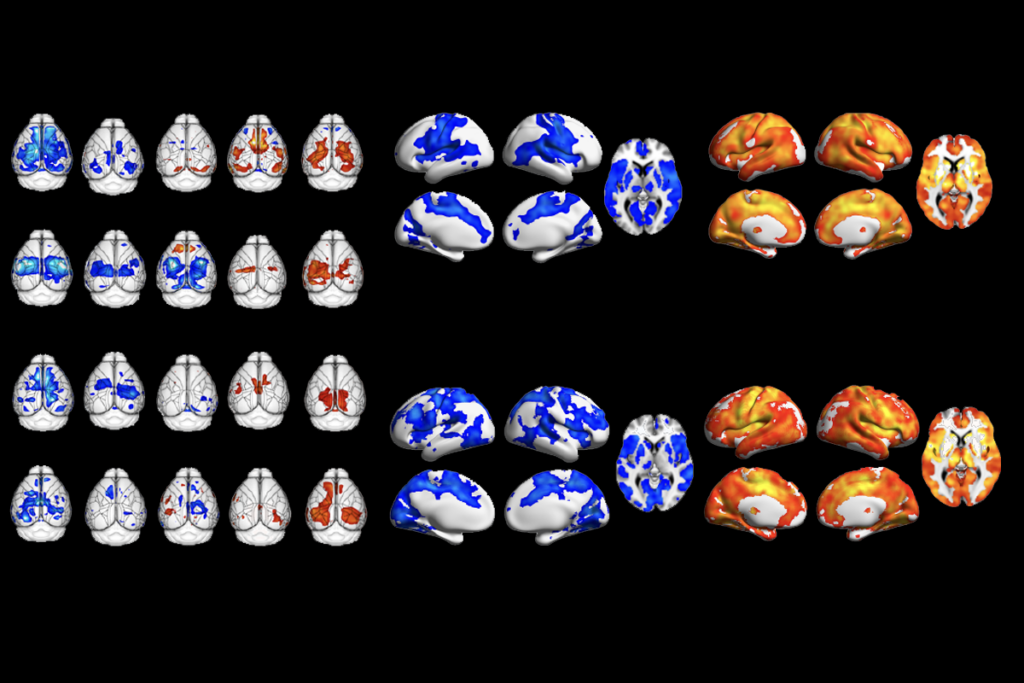
Too much or too little brain synchrony may underlie autism subtypes
Explore more from The Transmitter
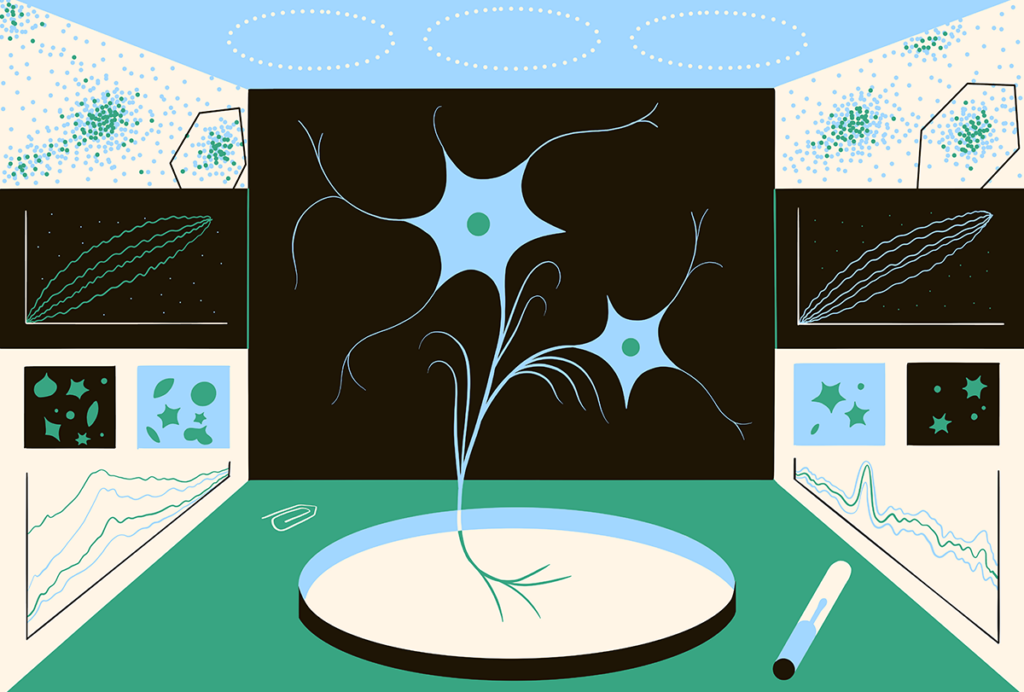
This paper changed my life: Shane Liddelow on two papers that upended astrocyte research
Dean Buonomano explores the concept of time in neuroscience and physics
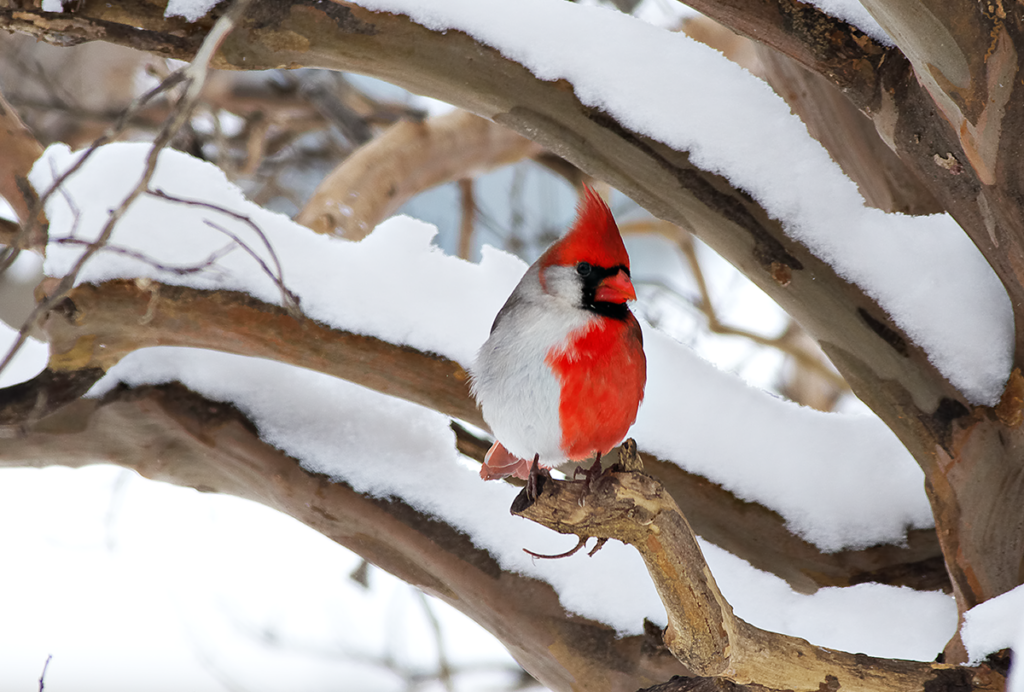