Big brains may hold clues to origins of autism
The brain enlargement seen in many children with autism may reveal hints about the condition’s causes.
A persistent challenge to improving our understanding of autism is the fact that no single neurological feature unites all — or even a majority — of cases. For instance, in the probably thousands of studies in which researchers tried to identify neuroanatomical changes specific to autism, only one feature has emerged with any consistency: an unusual enlargement of the brain in early childhood.
Up to 20 percent of children with autism have early brain overgrowth1, 2. This enlargement usually becomes noticeable a few months after birth and persists until late childhood or early adolescence, when growth slows and the average brain size of typically developing children catches up.
Two questions come up when contemplating this enlargement: First, what is causing it? And second, how is it linked to the core features of autism?
Answering these questions holds considerable promise for autism research and possibly for addressing the needs of affected children. For instance, understanding the genetic or environmental causes of brain overgrowth could aid early diagnosis and potentially lead to ways of preventing changes in the brain in autism, as this phenomenon predates the first behavioral signs in affected children.
Importantly, recognizing how structural brain differences can lead to behavioral changes in autism can help us to causally connect changes in certain brain areas, neuronal circuits and cells to autism. These insights may help us pinpoint the neurobiological changes that underlie autism more generally.
Debatable data:
Early brain overgrowth has been somewhat controversial as a defined feature of autism. Some studies in this area relied on comparing the head circumference of children who have autism with that of children in the general population. Methodological problems dogged many of these investigations, which often included control groups that differed in important ways from the affected children. In some cases, for example, when researchers recruited controls from the same communities as the children with autism, they found less pronounced differences in head circumference3.
The gap in head size between affected and unaffected children also diminished when scientists took the ancestry, sex, height and weight of the children into account. Autism cases associated with mutations leading to smaller head size further complicate the picture.
Still, magnetic resonance imaging (MRI) data, which can directly reveal size discrepancies in the brain, now indicate that a portion of children on the autism spectrum do show brain enlargement compared with age-matched controls2, 4-6.
Studies of animals with mutations in autism risk genes have tied those mutations directly to brain enlargement. These studies have established early brain overgrowth as a key feature of a subset of autism cases.
Cell surplus:
Most of the brain enlargement seen in children with autism occurs in the cerebrum, the intricately folded surface of the brain and the fibrous connections beneath it. The bumpy surface of the brain, called the cerebral cortex, is of particular interest to autism researchers, as it is home to higher brain functions such as attention, perception, cognition, language and consciousness.
Researchers have gone to considerable effort to discern whether overgrowth occurs predominantly in the gray matter, which is home to neuronal cell bodies, or the white matter, which consists of nerve projections.
Work in children with mutations in the autism-linked gene PTEN suggests that white matter — particularly the bridge of axons connecting the left and right hemispheres, called the corpus callosum — bears the brunt of the overgrowth. But most studies have found gray matter in the cerebral cortex to be the main culprit, particularly in young children.
A key study of cerebral gray matter in postmortem brains revealed that children with autism who show early brain overgrowth have 67 percent more neurons in the prefrontal cortex than controls do7.
What’s driving this increase in neurons is unclear, but several studies point to the abnormal proliferation of neural progenitors, immature cells that give rise to neurons. Between weeks 7 and 20 of gestation, these precursor cells divide rapidly, giving birth to hundreds of thousands of neurons every minute. Ultimately, they yield roughly 100 billion nerve cells.
Postmortem brain tissue from children with autism shows disruptions in the organization of layers of cells in the cortex8. This work lends additional support for the concept that cells proliferate abnormally in these children.
Regional differences:
Studies in animal models of autism also support a central role for excess proliferation of neurons in early brain overgrowth.
For instance, a 2012 study in zebrafish and mice implicates KCTD13, a gene that resides in the autism-linked chromosomal region 16p11.2, in brain enlargement9. Specifically, deletion of the gene in zebrafish embryos produces an enlarged head in the fish as it does in people. Interfering with KCTD13 function in mouse embryos also boosts neural proliferation in the mice.
Similarly, blocking expression of the autism gene CHD8 in zebrafish embryos boosts the number of neural progenitors in the forebrain and midbrain10, 11. Mice with mutations in another autism-linked gene, WDFY3, also have too many neural progenitors and abnormal layering in the cortex12.
Neurogenesis before birth occurs with different timing and at different rates in different brain regions. This regional variability could provide clues about how the process goes awry.
Imaging studies show that early brain overgrowth tends to affect the frontal and temporal lobes (front and sides) of the cerebral cortex more than its parietal and occipital lobes, which sit toward the rear of the head13. The frontal and temporal lobes are involved in the complex behaviors that are predominantly affected in autism.
For example, these lobes contain Broca’s and Wernicke’s areas, which are central to language production and perception. The orbitofrontal cortex, the ventrolateral prefrontal cortex, the superior temporal sulcus and the insula play key roles in social behavior and emotional processing. Alterations in these areas may underlie many of the behavioral characteristics of autism.
Inflammatory finding:
Early brain overgrowth could stem from environmental factors. Multiple studies have hinted at a link between inflammation in the womb and atypical brain development.
For instance, pregnant women may produce antibodies that are directed against the fetus and target factors in the developing brain that control how neural progenitor cells multiply and mature.
Cerebral overgrowth may also cause changes in the proportions of different cell types in the affected brain areas. It might, for instance, lead to an abundance of excitatory neurons relative to inhibitory neurons in the cerebral cortex. Indeed, postmortem brain studies have identified individuals who have too few of a special type of interneuron that expresses the molecule parvalbumin.
Understanding the nature of early brain overgrowth can provide key insights into its effects on brain circuit formation and behavior. It may also lead to biomarker-based diagnostics for autism.
Progress in this area will depend on improved imaging technologies, new animal models and access to brain tissue from individuals with autism.
Konstantinos Zarbalis is assistant professor of pathology and laboratory medicine at the University of California, Davis and assistant investigator at the Shriners Hospital for Children in Sacramento, California.
References:
- Fombonne E. et al. J. Autism Dev. Disord. 29, 113-119 (1999) PubMed
- Nordahl C.W. et al. Proc. Natl. Acad. Sci. USA 108, 20195-20200 (2011) PubMed
- Raznahan A. et al. Biol. Psychiatry 74, 563-575 (2013) PubMed
- Courchesne E. et al. Neurology 57, 245-254 (2001) PubMed
- Sparks B.F. et al. Neurology 59, 184-192 (2002) PubMed
- Courchesne E. et al. JAMA 290, 337-344 (2003) PubMed
- Courchesne E. et al. JAMA 306, 2001-2010 (2011) PubMed
- Stoner R. et al. N. Engl. J. Med. 370, 1209-1219 (2014) PubMed
- Golzio C. et al. Nature 485, 363-367 (2012) PubMed
- Bernier R. et al. Cell 158, 263-276 (2014) PubMed
- Sugathan A. et al. Proc. Natl. Acad. Sci. USA 111, E4468-4477 (2014) PubMed
- Orosco L.A. et al. Nat. Commun. 5, 4692 (2014) PubMed
- Courchesne E. et al. Brain Res. 1380, 138-145 (2011) PubMed
Recommended reading
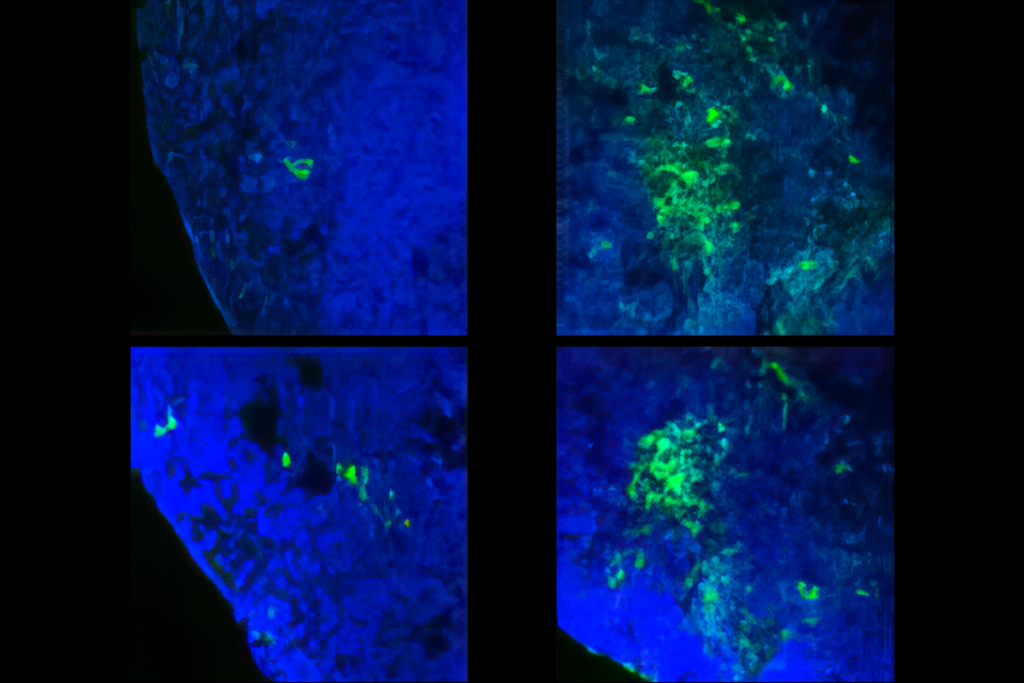
Personalized medicine; astroglia organoids; fast track for fragile X drug
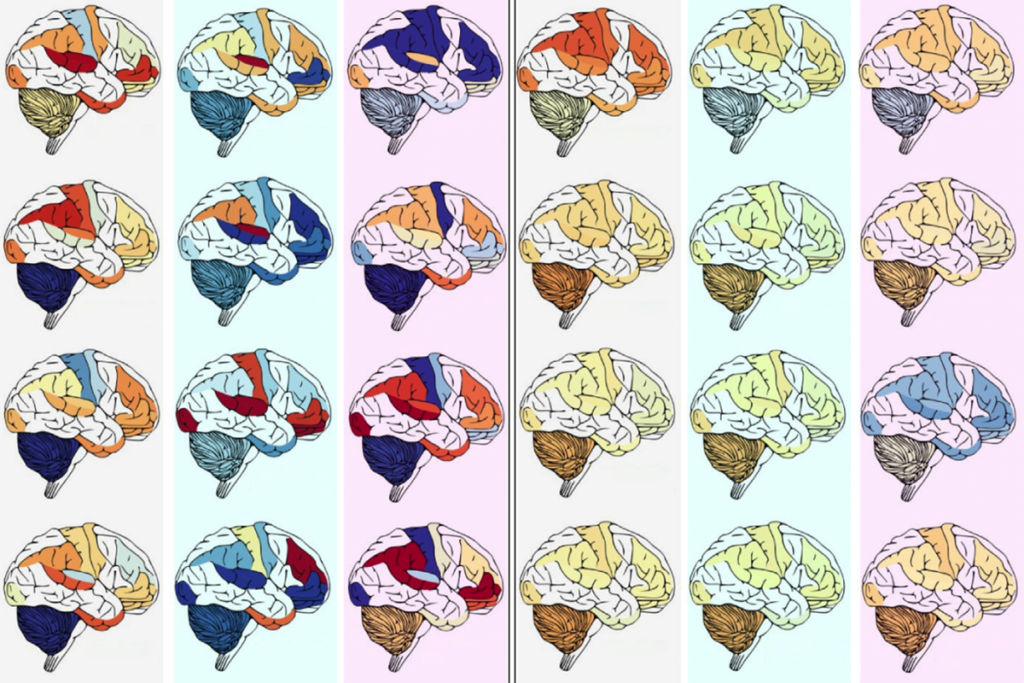
X marks the spot in search for autism variants
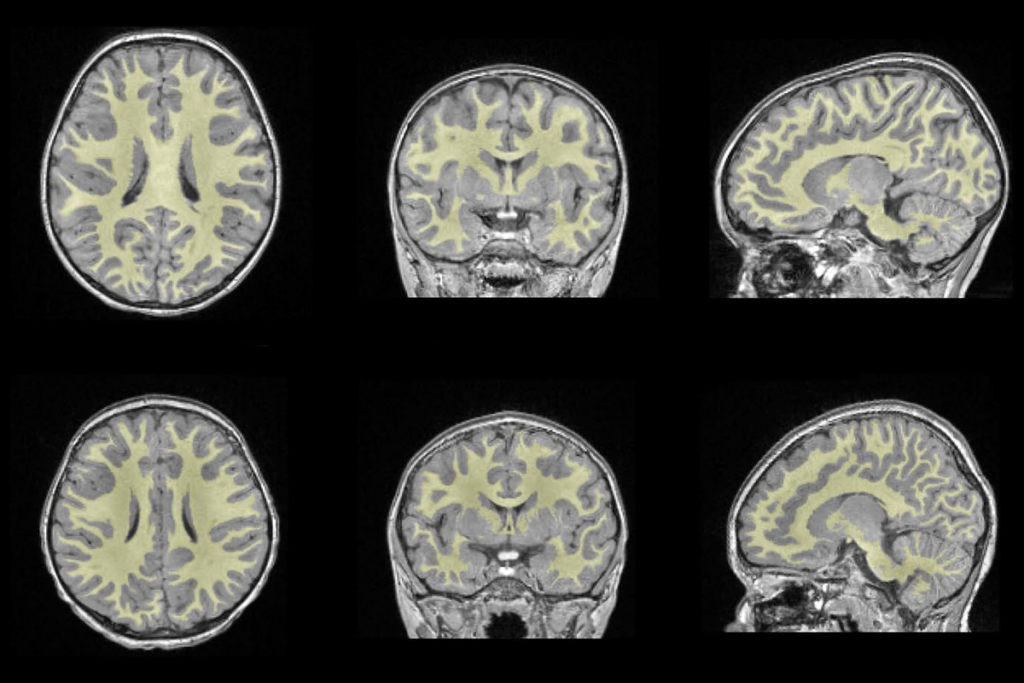
White-matter changes; lipids and neuronal migration; dementia
Explore more from The Transmitter
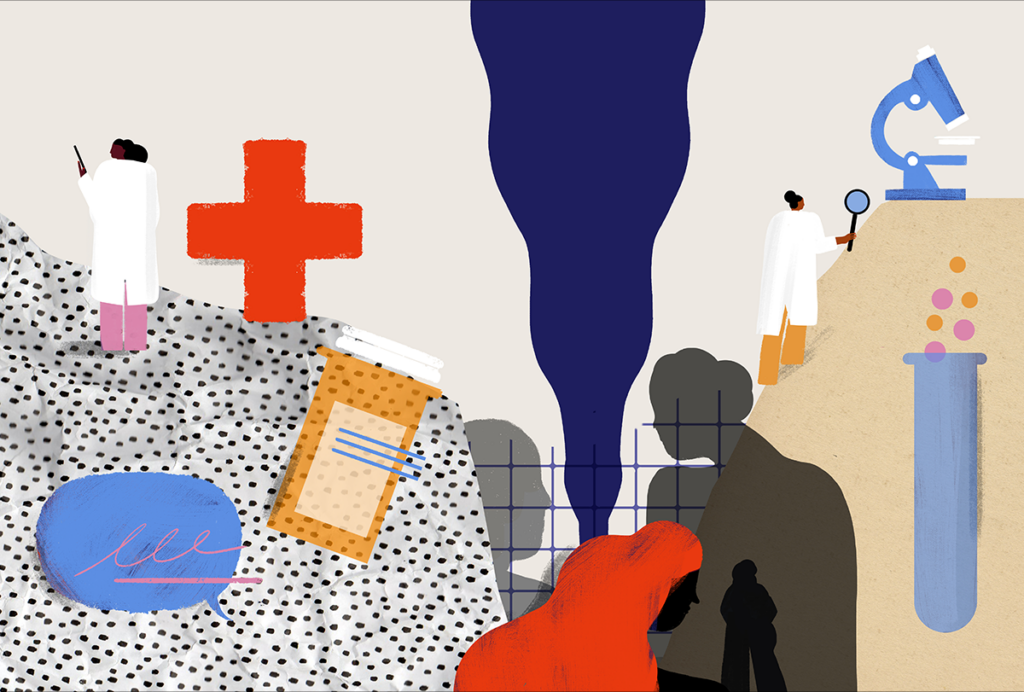
Neuroscientists need to do better at explaining basic mental health research
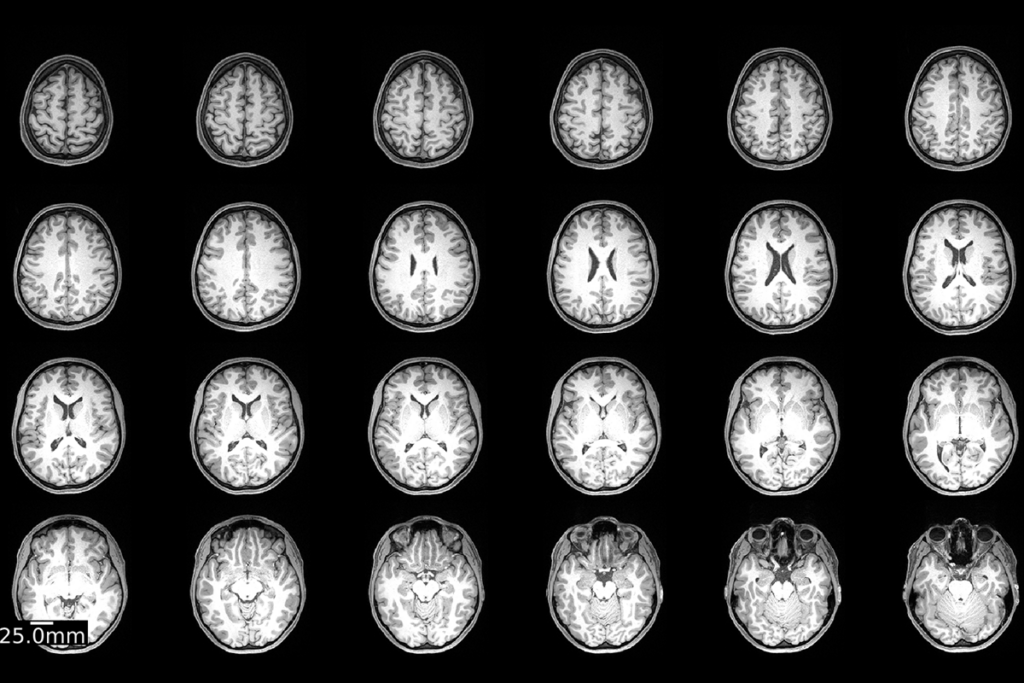
Dose, scan, repeat: Tracking the neurological effects of oral contraceptives
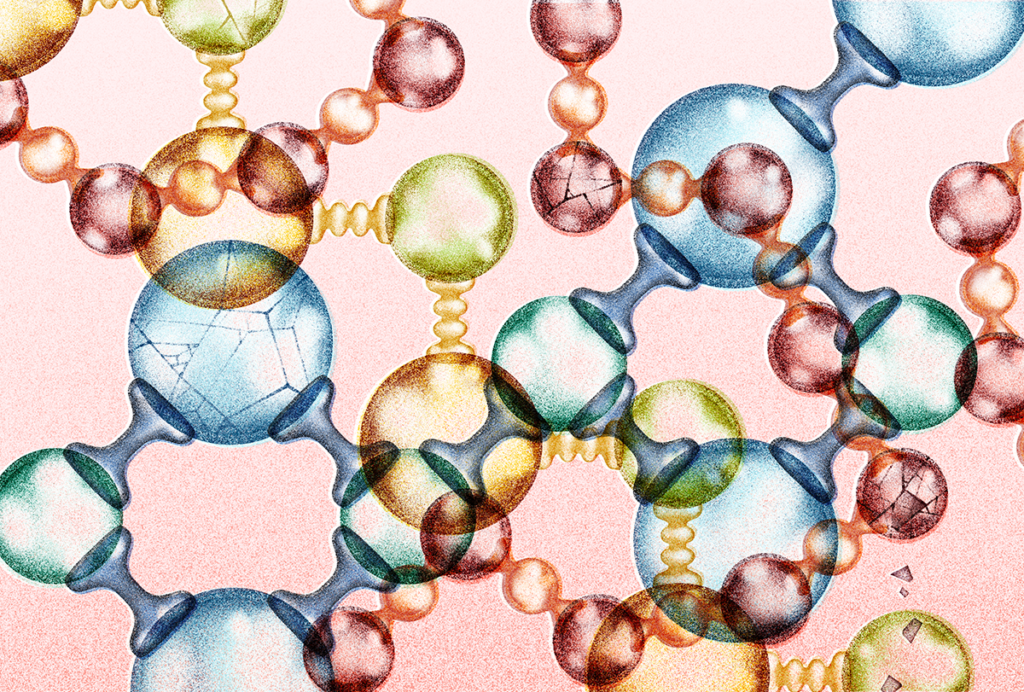