Angelman syndrome gene tied to brain’s adaptability
A gene linked to autism and other developmental disorders can undermine the brain’s ability to change the strength of connections between neurons.
A gene linked to autism and other developmental disorders can undermine the brain’s ability to change the strength of connections between neurons, according to a study published 10 May in Nature Neuroscience1.
The work adds to the growing body of evidence showing that these disorders prevent the brain from adapting to new experiences, and may provide new options for pharmacological or behavioral therapies, say the researchers.
People with mutations in the UBE3A gene develop Angelman syndrome, a disorder marked by stunted language and cognitive development. Children with Angelman syndrome stop passing developmental milestones, such as acquiring new words, between 12 and 18 months old.
That’s the same critical period in which the developing brain undergoes synaptic plasticity — the cellular learning process whereby sensory stimuli strengthen or weaken synapses, the junctions between neurons.
Lead investigators Benjamin Philpot, of the University of North Carolina at Chapel Hill School of Medicine, and Michael Ehlers, a neurobiologist at Duke University, thought that timing might not be a coincidence. Neither scientist had previously studied Angelman syndrome, but their mutual interest in synapse modeling led them to this unique single-gene disorder.
Developmental defects in synaptic circuits — the networks of neuronal connections designed to process specific bits of information — have been implicated in neuro-developmental disorders such Angelman syndrome and Rett syndrome, a genetic disorder that causes mental retardation and seizures2. But no one knew what caused these brain circuits to break down during development.
Angelman syndrome is caused by the deletion of the maternal copy of UBE3A3. Mutations of this gene have also been implicated in autism4. What’s more, the region of chromosome 15 that contains the UBE3A gene is the site of the most common genetic variant associated with autism: a duplication that leads to over-expression of UBE3A5.
The UBE3A enzyme produced by the gene is part of a pathway that keeps proteins expressed at proper levels. If a protein is over-expressed, UBE3A tags it to be destroyed.
Philpot and Ehlers hypothesized that UBE3A’s role in protein expression is necessary for synaptic plasticity in the neocortex, the area of the brain responsible for learning and memory.
To test this idea, the scientists took advantage of the Angelman mouse model, which doesn’t make UBE3A. They focused on a part of the animal’s brain where experience-dependent activity has been studied in detail: the visual cortex.
Blind mice:
Mice are born with their eyes closed. For the first two weeks of life, the animal’s visual cortex develops without visual experience. After that period, the eyes open, letting in light and causing a dramatic reorganization of brain circuits in the region. The new sensory input causes a profound change in the number of synapses and triggers the brain to form new circuits, says Ehlers.
In their first experiments, the team measured nerve impulses in brain slices from the visual cortex of normal mice and Angelman syndrome mice that had just opened their eyes. They found that the Angelman mice have dramatically less neuronal activity than the normal mice do. That suggests that the reorganization of synapses in response to visual experience does not occur in the Angelman mice, Ehlers says.
In a second batch of tests, the scientists raised normal and Angelman mice in either pure light or pure darkness during the two-week critical period following eye-opening. In normal mice, this sensory deprivation leads to less neural activity than do light conditions. In contrast, Angelman mice show no difference in synaptic maturation whether raised in light or dark.
“This kind of synaptic rigidity is normal in animals, but it usually happens much later in life,” says Ehlers.
Surprisingly, the scientists did produce plasticity in the Angelman mice by depriving the animals of light after the critical period. That is, Angelman mice maintain a dormant ability to express synaptic plasticity.
As a final test, the researchers shut one eye from each mouse shut at three weeks of age. When normal mice are deprived of vision in one eye, their brain structure gradually changes to rely on the other eye for vision processing, a classic example of how experience modifies the brain during this critical period. No such adaptation was found in the brains of the visually impaired Angelman mice.
Taken together, the experiments show that large-scale remodeling of the cortex does not happen in Angelman mice, but that some forms of synaptic plasticity can be recovered with sensory manipulation.
“Our work demonstrates the molecular machinery is still in place to express plasticity in Angelman syndrome mice,” says Philpot. “We simply have to find a viable behavioral or pharmacological manipulation to tap into this dormant ability,” he says.
Saving synapses:
These new findings are the third example of restoring synaptic plasticity in a mouse model of an autism-related disorder. Scientists have also done it in mouse models of Rett syndrome and fragile X syndrome, an inherited form of mental retardation and a well-known cause of autism.
Interestingly, all three examples make use of different therapeutic approaches to restore plasticity.
In 2007, Mark Bear, professor of neuroscience at Massachusetts Institute of Technology (MIT), and colleagues were able to recover synaptic plasticity in a mouse model of fragile X syndrome. The team engineered the mice to produce half of the normal level of mGluR5, a neurotransmitter receptor whose excessive signaling may lead to fragile X syndrome6. Bear’s group is now exploring drugs that may restore plasticity by reducing the activity of mGluR5 receptors.
In February 2009, Mriganka Sur‘s team demonstrated that synaptic plasticity could be stabilized in mouse models of Rett syndrome by treating the animals with insulin-like growth factor 1, a protein known to promote synaptic maturation7.
“What is impressive about the overlap between these single-gene disorders is that downstream from the gene, they all have an impact on synapse maturation and plasticity,” says Sur, professor of neuroscience at MIT.
This mechanistic understanding may provide a number of potential drug targets or behavioral therapies, he adds. “Now that we understand how these genes disrupt the synapse, there are many ways we can intervene to restore the synaptic circuits.”
Whether these approaches will offer a treatment option for autism remains to be seen. “To the extent that the complex forms of autism have synaptic dysfunction at their core, some of these ideas may well hold,” says Sur.
References:
- Yashiro K. et al. Nat. Neurosci. Epub ahead of print (2009) PubMed
- Zoghbi H.Y. Science 302, 826-830 (2003) PubMed
- Rougeulle C. et al. Nat. Genet. 17, 14-15 (1997) PubMed
- Schroer R.J. et al. Am. J. Med. Genet. 76, 327-336 (1998) PubMed
- Glessner J.T. et al. Nature Epub ahead of print (2009) PubMed
- Dölen G. et al. Neuron 56, 955-962 (2007) PubMed
- Tropea D. et al. Proc. Natl. Acad. Sci. U. S. A. 106, 2029-2034 (2009) PubMed
Recommended reading
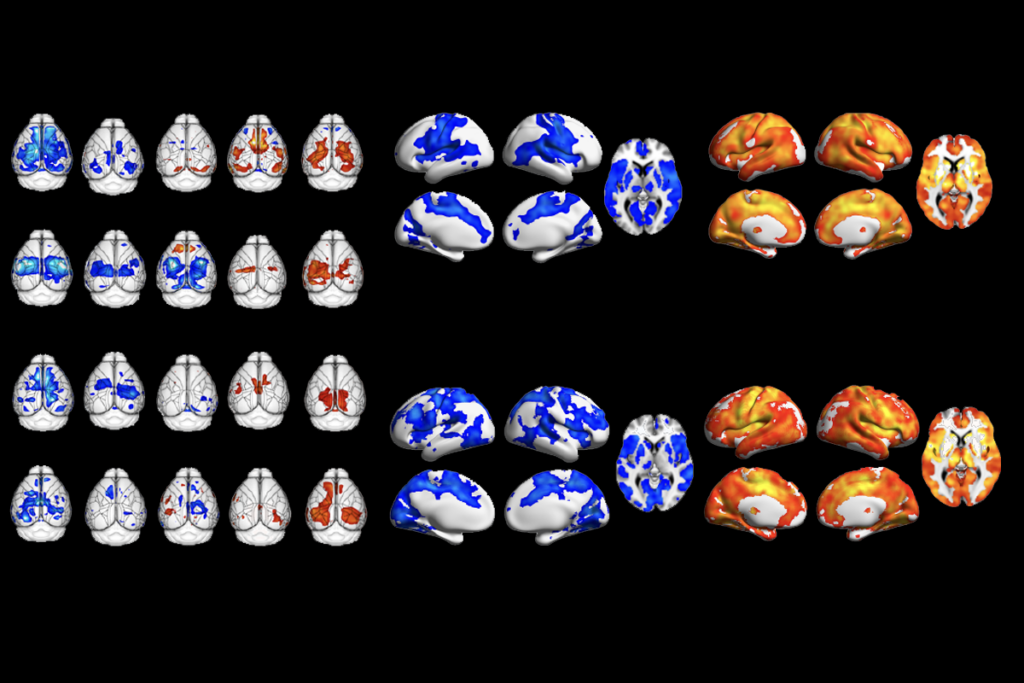
Too much or too little brain synchrony may underlie autism subtypes
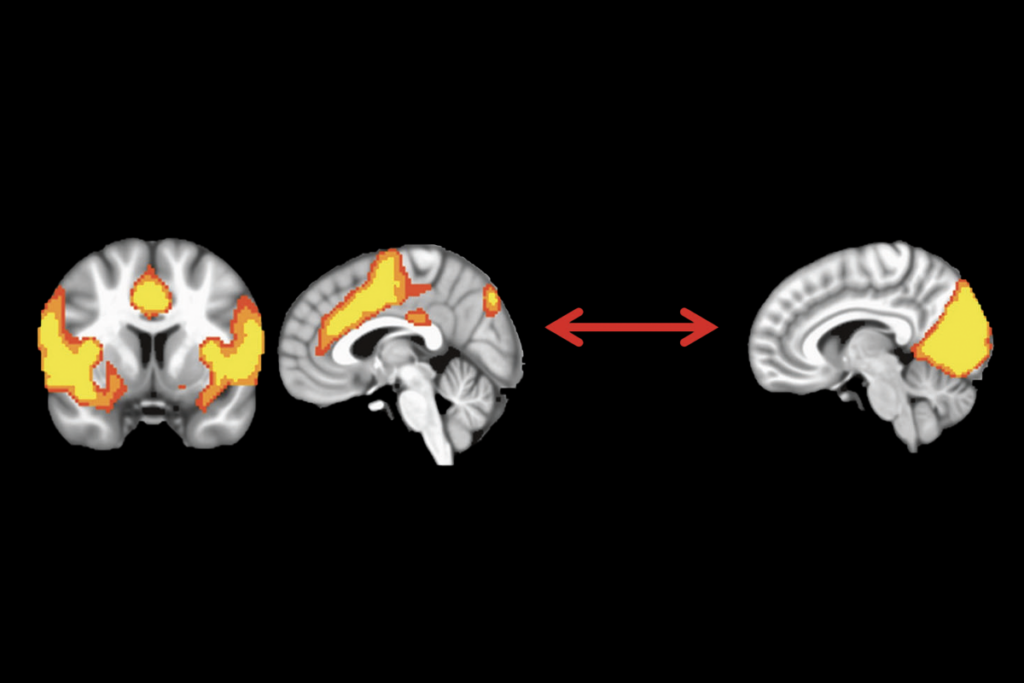
Developmental delay patterns differ with diagnosis; and more
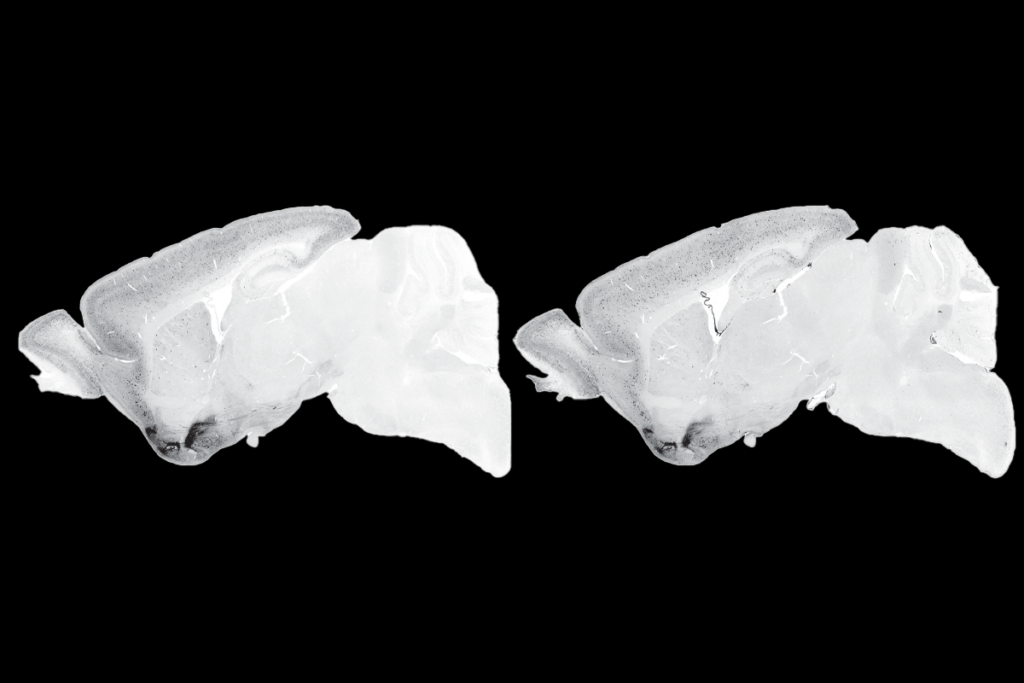
Split gene therapy delivers promise in mice modeling Dravet syndrome
Explore more from The Transmitter
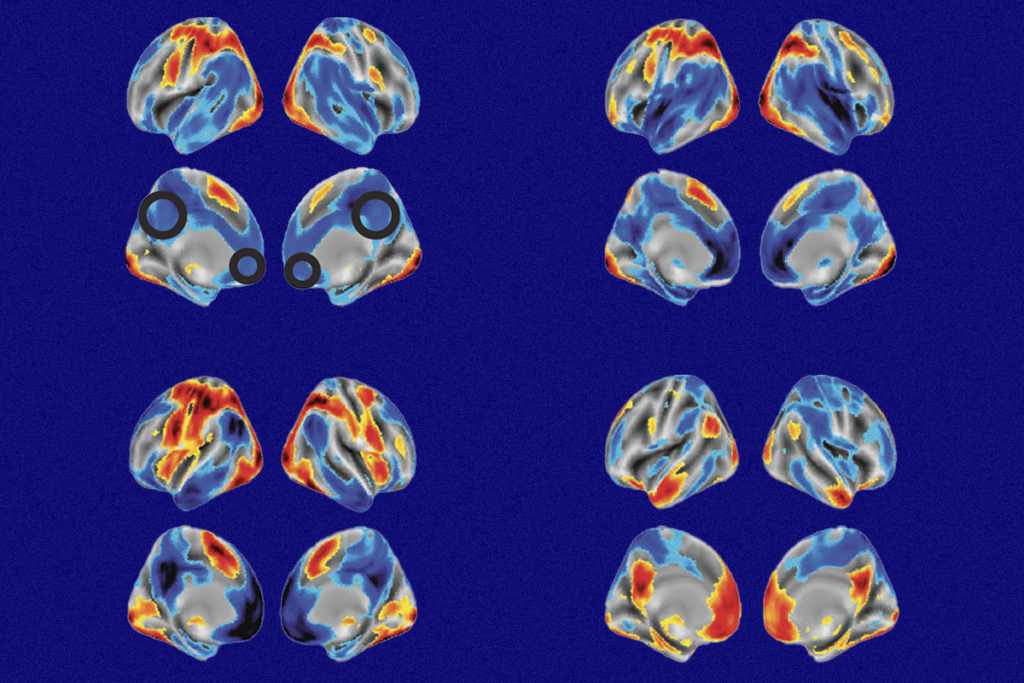
During decision-making, brain shows multiple distinct subtypes of activity
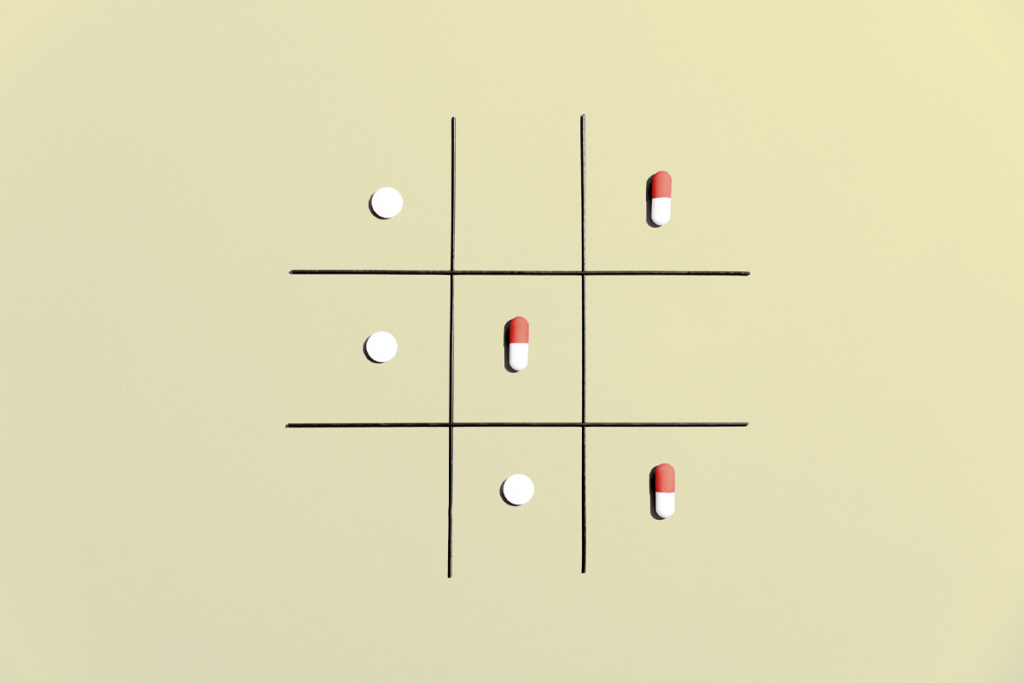
Basic pain research ‘is not working’: Q&A with Steven Prescott and Stéphanie Ratté
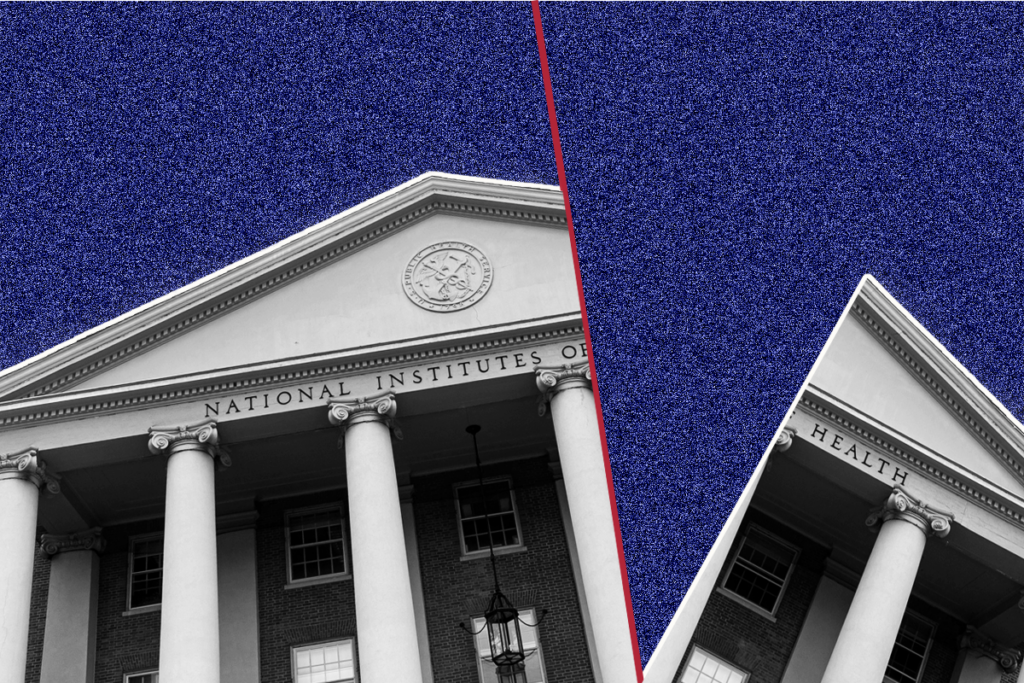