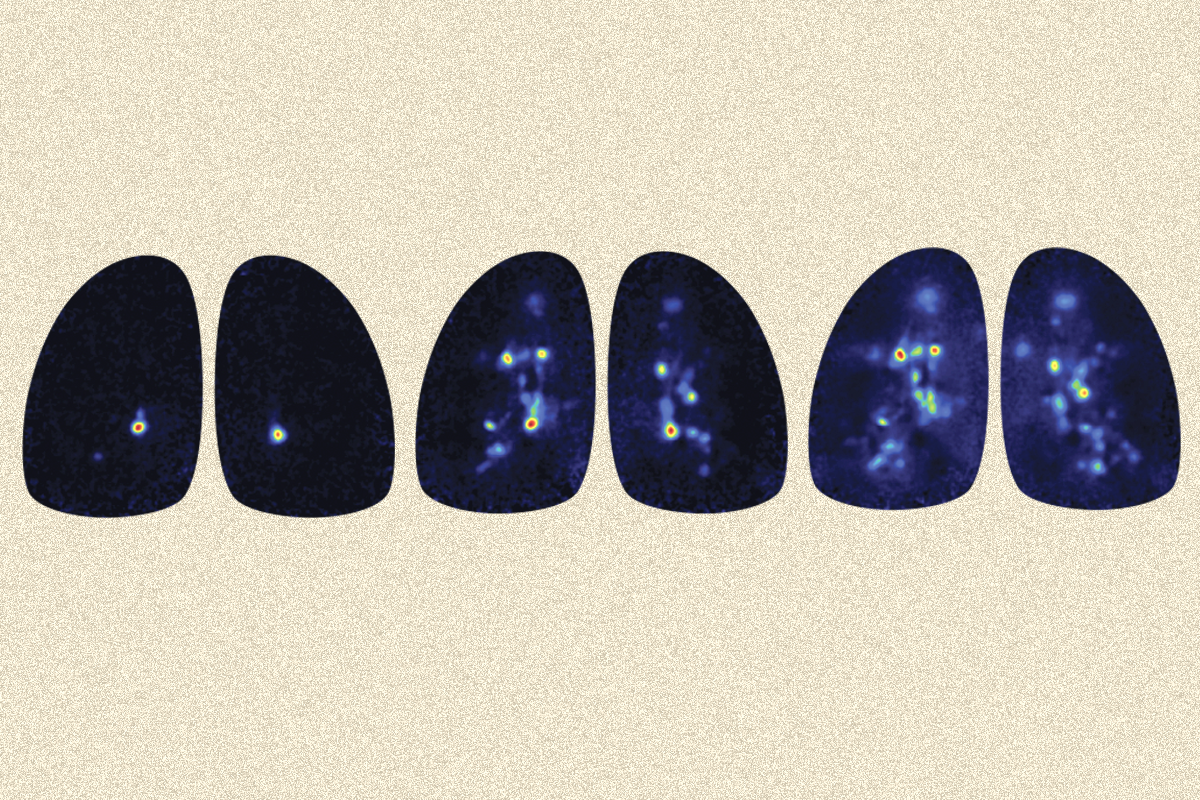
Smell studies often use unnaturally high odor concentrations, analysis reveals
It’s time to fashion olfactory neuroscience stimuli based on odor concentrations in the wild, say study investigators Elizabeth Hong and Matt Wachowiak.
Olfactory neuroscientists have known for a while that their stimuli stink.
In many experiments, lab animals inhale puffs of a single strong odorant for only a few seconds at a time, an experience that “likely bears little resemblance—either in concentration or in form—to the ways in which animals naturalistically interact with odors,” says Sandeep Robert Datta, professor of neurobiology at Harvard University. In the wild, animals sniff subtle plumes of various, often comingled, odors wafting through the air.
Even though the field knew about this mismatch, “we have our hands tied behind our back because it’s very difficult to measure odors in natural settings,” says David Coppola, retired professor of biology at Randolph-Macon College. And as a result, researchers lacked a dataset of natural concentrations of odor molecules, or odorants, to use as a reference for their experimental stimuli, says Elizabeth Hong, professor of neuroscience at the California Institute of Technology.
So Hong and Matt Wachowiak, professor of neurobiology at the University of Utah, set about making one. They pored through the literature from the fields of food science, environmental toxicology, atmospheric chemistry and others to assemble a collection of odorant concentrations in different settings, including a pine forest, a pig farm, a composting bioreactor and a cannabis grow room. A majority of the data came from “headspace” studies that measure odorants emanating from food or other fragrant items enclosed in a jar.
Their effort revealed the scale of the problem: Most of the odor sources they compiled have natural concentrations in the range of parts per billion, whereas olfaction experiments use concentrations on the scale of parts per million, according to their paper published 5 March in The Journal of Neuroscience. The difference in scale is like a drop of water in a 10-gallon home aquarium compared with a drop of water in a 10,000-gallon swimming pool.
“We are learning about the sense of smell from a relatively unphysiological experimental setting,” says Marc Spehr, professor of chemosensation at RWTH Aachen University, who was not involved in the work. It is akin to audition researchers attempting to study the auditory system only “by putting your subjects next to a jet plane.”
Studies that use strong stimuli have gleaned important insights about the olfactory system, Hong says. But because different concentrations of odors activate olfactory brain areas in different ways, it’s time to take the complexity and concentration of odors into account to answer more nuanced and mechanistic questions, she adds.
“For the evolution of the field, this will be important,” Spehr says.
A
n animal’s sense of smell begins when odor molecules bind to the receptors of neurons located in the nasal epithelium. These neurons then form synapses with neurons in the olfactory bulb. Clusters of these synapses form spherical structures called glomeruli, each of which receives input from only one receptor type. Multiple odorants can bind to each receptor, and in turn, each odorant can bind to multiple receptors, with varying affinities. The brain decodes an odor’s identity from its unique pattern of activated glomeruli, or so researchers hypothesize.But subtle scents act differently: At low concentrations, in the parts-per-billion range, about 25 percent of glomeruli respond to only one odorant apiece, Wachowiak reported in 2022. This level matches the environmental concentrations Wachowiak and Hong cataloged in their review and falls within the range of concentrations rodents can detect in tests.
Often, then, “the olfactory system is functioning at a near-threshold level,” Wachowiak proposes. “That’s changed the way I think about the question of, ‘What’s the olfactory system doing for the animal, and how is it being used?’” The data compiled in the review suggest the “most common operating regime of the system is just trying to detect an odor in the first place,” he says.
Datta says he agrees that experimental concentrations are likely too high and that mice are capable of detecting concentrations at the edge of their abilities, “but that’s not the same as arguing that in the natural world, under most biological circumstances, that’s what the system is doing.”
Work from Datta proposes a different framework. Olfactory sensory neurons in the nose express a selection of activity-dependent genes whose expression changes based on what an animal smells—and for how long. In a 2021 paper, Datta predicted which of three differently scented cages a mouse had spent the past few hours in based on its olfactory sensory neuron transcriptome. “What it means is that, under normal circumstances, your nose is always contending with enough odor to activate every channel differentially,” Datta says. “So instead of living in a world where odors are fundamentally sparse, our data argue that you live in a world where odors are fundamentally dense” and distracting.
Plus, some environments, such as a mouse burrow, probably do contain high concentrations of certain odors. The olfactory system “is capable of all sorts of things and probably deals with dense and sparse all at once, all the time,” Datta says. Either way, “we should be aware of the concentrations we’re using and the ways in which what we do reflects nature.”
P
art of the reason that this methodological hang-up exists stems from the nature of odors: It is really hard to measure the concentration of an odorant when it is in vapor form. “I won’t call it a nightmare, but close to it,” Spehr says.It is much simpler to measure auditory or visual stimuli. “With your iPhone, you can go out and probably get an app and measure light intensity and sound intensity,” Wachowiak says, whereas measuring odor intensity requires “very expensive, sophisticated” pieces of chemistry equipment.
Plus, odorants travel in plumes, so some pockets of air have higher concentrations than others; standard measurement methods cannot capture this variance or dynamism. Even if you measure the rough concentration of an odor in a space, it’s difficult to capture the exact concentration an animal sniffs as it moves around, says Tobias Ackels, group leader at the University of Bonn, who was not involved in the study.
As a result, investigators often don’t report their stimuli in a way that makes it possible to determine the exact odorant concentrations—that’s the case for about one-third of rodent studies and 80 percent of insect studies, Hong and Wachowiak found in their review. But there is a feasible first step for the field, they say: Researchers can use the vapor pressure of a given odor to calculate its approximate concentration.
“It’s really not very precise,” Spehr says of that approach, “but it’s something that gives you a ballpark information of what could be the concentration in the air.”
Another potential solution is to use natural odor sources in lieu of monomolecular odors from a chemical company. This works for some research questions, such as general features of the olfactory system that are agnostic to an odorant’s identity, but not for questions about “dose dependence, molecular feature detection and similar things,” Spehr says.
Researchers can also create blends of monomolecular odors that mimic natural odor sources, Hong says. “These types of stimuli will complement one another.”
The concentration database built from the literature of other fields is a “heroic effort,” says Coppola, who was not involved in its creation. But the settings included and the headspace studies are not adequate replacements for measurements from the environments animals actually inhabit, such as a mouse burrow, he says. “We need to go out in the field, where these behaviors are actually happening, and measure some concentrations of important molecules.”
In the meantime, the database is still a helpful starting point for researchers who want to calibrate their concentrations to a more appropriate level, Ackels says.
Overall, the review is “super important,” Datta says. “I think we do need to change our perspective, to think more about the naturalistic function of olfaction.”
Recommended reading
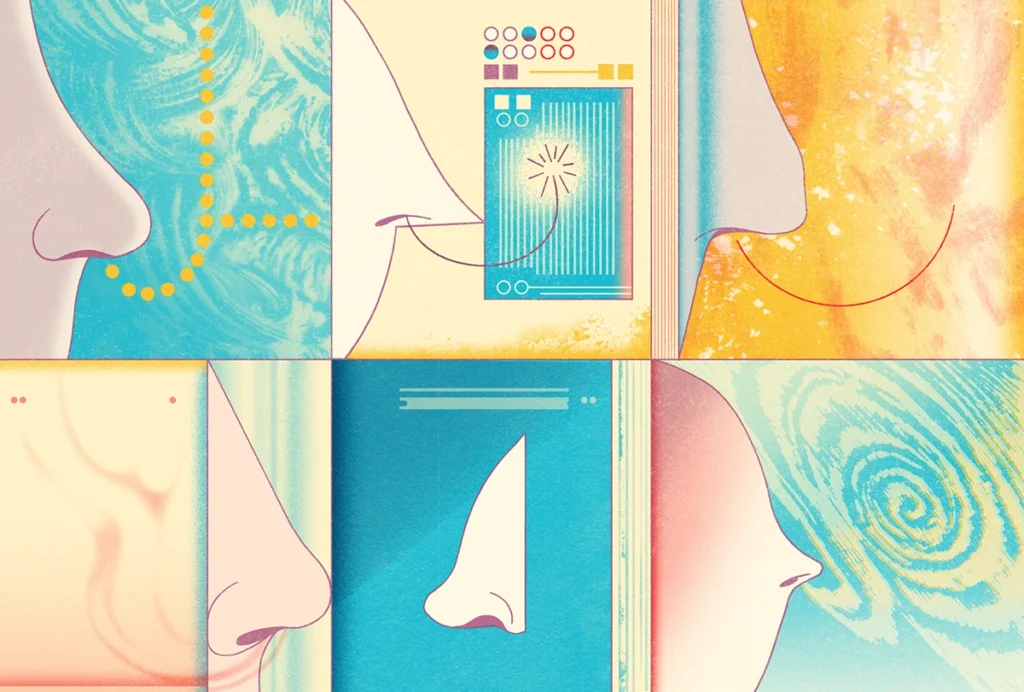
Consciously smelling—an edited excerpt from ‘Stinking Philosophy! Smell Perception, Cognition, and Consciousness’
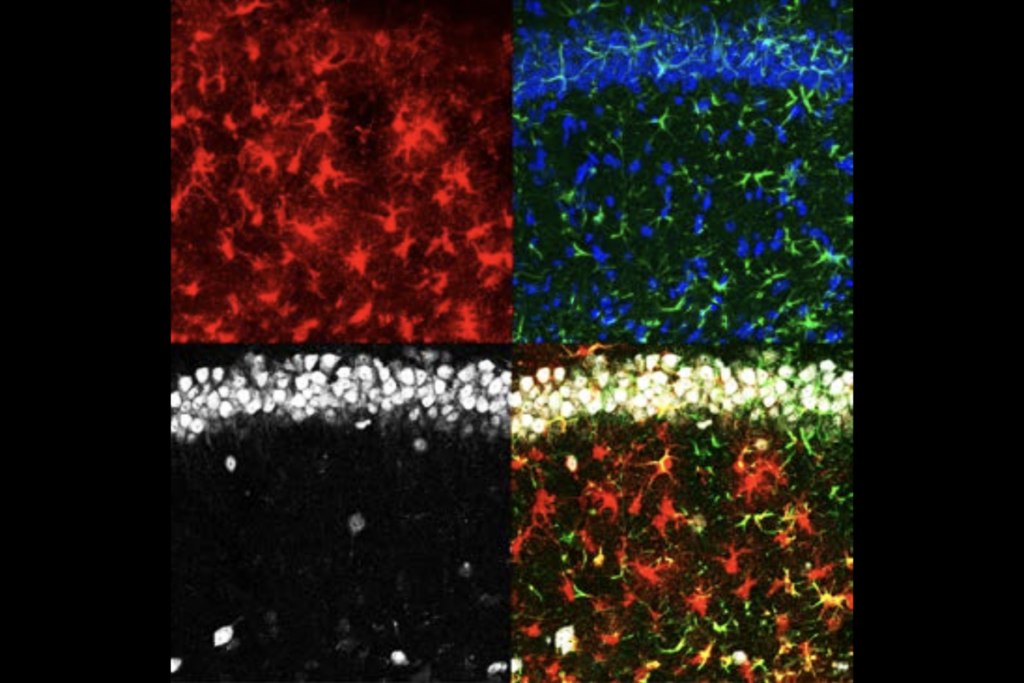
Astrocytes sense neuromodulators to orchestrate neuronal activity and shape behavior
Explore more from The Transmitter
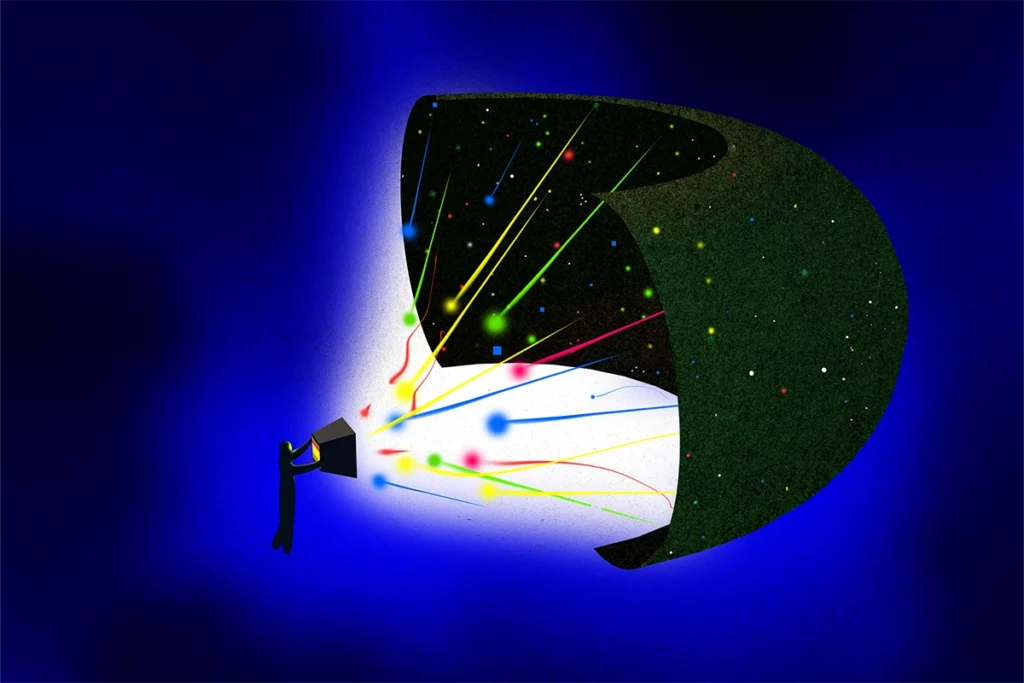
Imagining the ultimate systems neuroscience paper
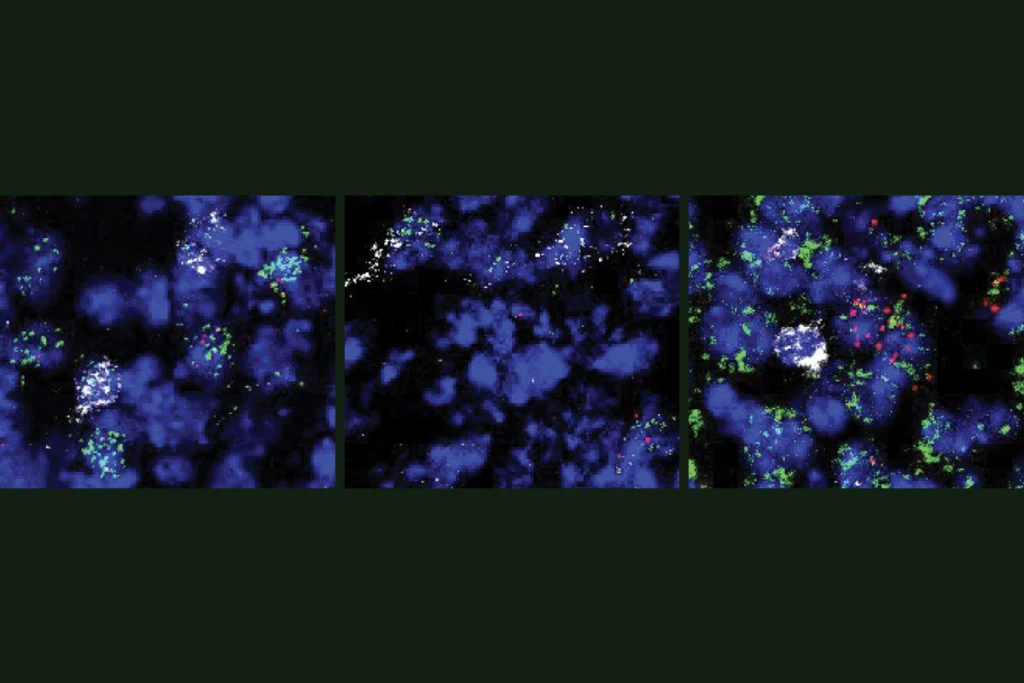
Novel neurons upend ‘yin-yang’ model of hunger, satiety in brain
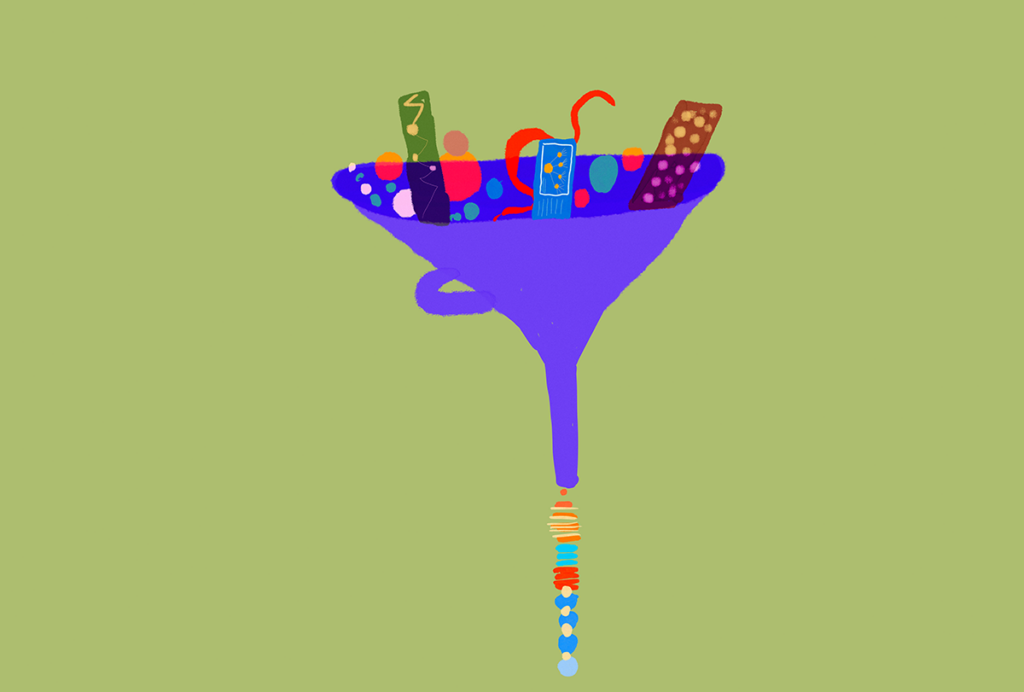