Organoids and assembloids offer a new window into human brain
These sophisticated 3D cultures reveal previously inaccessible stages of human brain development and enable the systematic study of disease genes.
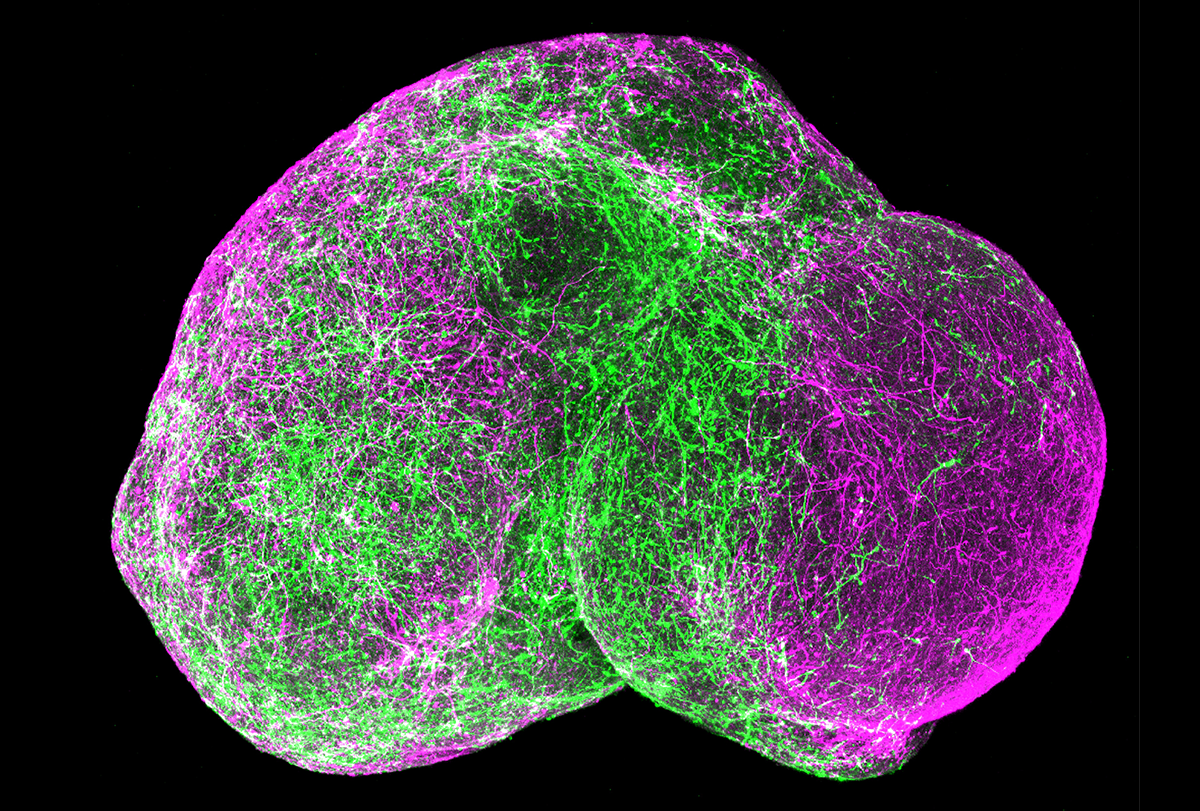
The unbearable inaccessibility of the human brain has been a major barrier to understanding both how the human nervous system assembles itself and how psychiatric and neurological disorders emerge. But thanks to new advances, it is becoming possible to access functional aspects of human brain development and function that were previously out of reach.
This progress has been driven primarily by advances in stem cell technologies, which make it possible to recapitulate developmental processes outside the human body. The journey began decades ago with the ability to grow stem cells in a dish, followed by the use of developmental signals to guide them into becoming neural cells. The field was truly catalyzed by the discovery of cell reprogramming and the democratization of stem cell technologies it enabled. Starting more than 15 years ago, my team and others began creating neurons from patients—initially rather inefficiently, but then with increasing ease as culture systems became more sophisticated. For example, cortical neurons derived from people with Timothy syndrome—a genetic form of autism and epilepsy caused by a mutation in a calcium channel present in excitable cells—revealed calcium deficits following depolarization. Some of these defects became more apparent when moving beyond traditional 2D preparations, such as when looking at the morphology of human neurons. For more than a decade, we and others have developed methods for growing these cells into more complex 3D structures, known as organoids, that mimic some of the structure and function of regions of the nervous system, offering a new window into human neurobiology and disease.
Giving cells this third dimension of freedom unleashes self-organization: Mirroring in-vivo development, organoids generate diverse neural and glial cell types, starting from radial glia to intermediate progenitors, deep and superficial layer neurons and then astrocytes. These organoids can be maintained in vitro for years. Fascinatingly, developmental timing in organoids is largely preserved. For example, neurons maintained in culture for about nine months can transition to a postnatal state simply by surviving long enough in the dish. This observation in organoids offers a fundamental insight into development: Brain cells have an intrinsic, species-specific developmental “timer.”
T
he nervous system harbors immense cellular diversity, partly because during early development it creates multiple niches with local cues that specify cell fates. These cells then migrate and connect across and outside the brain. To model this complexity, we combined different types of brain organoids into structures called assembloids, in which cells from different origins can intermingle. Forebrain assembloids, for example, integrate two types of organoids representing cortex and subpallial regions, where interneurons migrate and integrate into cortical microcircuits. In other assembloids, neurons form long-range connections, such as cortico-striatal assembloids in which cortical neurons connect with medium spiny neurons.More complex preparations combine three or four types of tissue, such as cortical organoids, spinal cord organoids and a clump of human muscle fibers. A sensory organoid containing neurons that sense noxious stimuli can be put in close proximity to spinal cord organoids, thalamic organoids and cortical organoids. Over weeks to months, neurons in these three- or four-part assembloids extend axons and connect with some specificity to other neurons. This gives rise to emergent properties, such as the contraction of human muscle under the control of deep-layer corticospinal neurons or the transmission of electrical signals from sensory neurons to cortical neurons after exposure to capsaicin or other noxious stimuli. Indeed, it is these emergent properties—interactions among parts brought up together—that distinguish assembloids from conventional co-culture systems or organoids.
This approach has yielded several insights. For instance, even with minimal instructions, cells connect with specific partners—cortical neurons project to striatal neurons, but not vice versa, for example. These systems can also integrate CRISPR-based approaches, making it possible to systematically map hundreds of disease genes to specific, previously inaccessible stages of human brain development, such as the migration of cortical interneurons and their role in establishing the excitation-to-inhibition balance in the cerebral cortex.
Importantly, disease modeling has revealed mechanisms underlying various conditions. Growing cortical neurons derived from individuals with Timothy syndrome into 3D organoids and assembloids, we found that interneurons with the Timothy syndrome variant don’t migrate properly and fail to integrate normally into cortical circuitry. This work represents the first time a novel potential therapy for a psychiatric disease was identified exclusively through stem cell models. (For the latest developments on this model, including efforts to develop new treatments, see “RNA drug corrects calcium signaling in chimeric model of Timothy syndrome.”)
T
here are, however, limitations to organoid and assembloid experimentation. Cytoarchitectural features, such as the layered structure of the cortex, remain challenging to model in vitro because of the absence of physical support, vascularization and other cues. Cells also struggle to achieve full maturation in vitro, rarely reaching the size or acquiring the electrical properties of in vivo neurons.In this context, transplantation into animal models—performed early in development, before the closure of critical periods—can enable more complete maturation and therapeutic testing directly on human neurons in vivo. More specifically, intact cortical organoids can be placed in the cerebral cortex of developing rodents, where they can grow to generate a “unit” of tissue with human neurons that receive inputs from whiskers (if placed in the somatosensory cortex) or can project to other brain regions and modulate animal behavior in reward and other tasks.
Interestingly, human cortical neurons grown in the rat cortex can grow six- to eight-fold larger than those maintained in vitro, which suggests culture systems lack nutrients, growth factors or electrical signals that are required for neurons to reach mature cell states. Tracking what happens to neurons after transplantation can reveal mechanisms in disease and be used to test therapeutics on human neurons in an in-vivo context, as my team recently did for Timothy syndrome.
The coming decade promises to be transformative for work in these model systems. As more labs adopt the models and the methodologies grow more sophisticated, we will capture additional processes of human brain assembly, maturation and perhaps even aging. These efforts are poised to uncover unique programs underlying the expansion of the human cortex, as well as mechanisms that regulate developmental timing in both in-vitro and in-vivo contexts. As we approach the limits of what can be modeled in vitro, we will learn how much of brain organogenesis is influenced by extrinsic factors outside the human body and how complex human circuits can be created in living organisms. Finally, these advances will accelerate the discovery of molecular and cellular phenotypes for disorders of development and degeneration, paving the way for a biologically informed classification of these conditions and the rational design of therapeutics.
Recommended reading
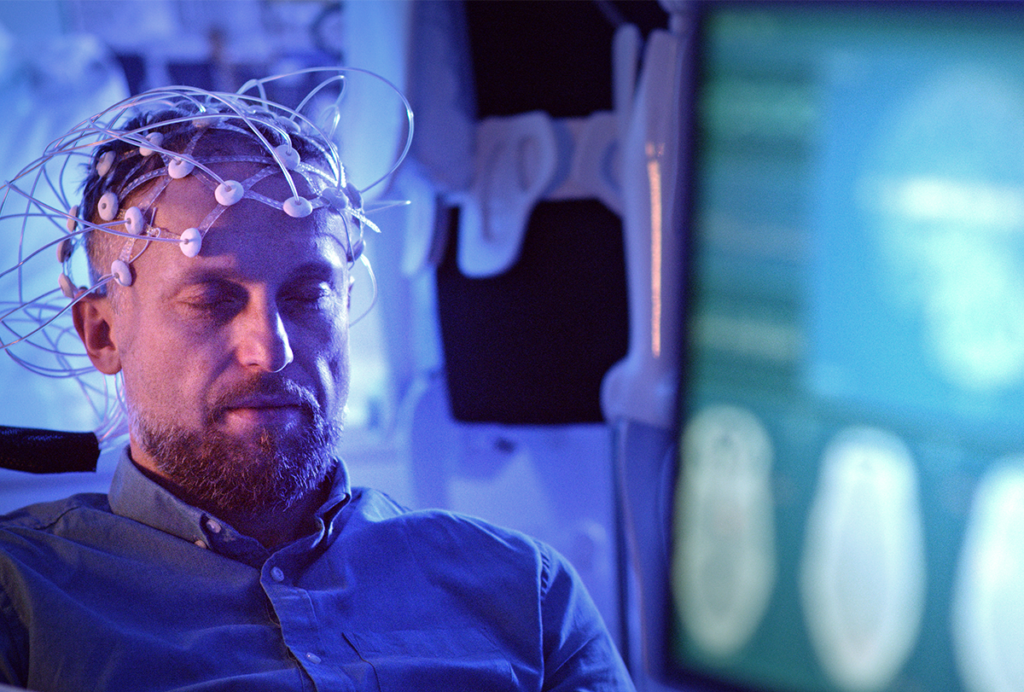
Noninvasive technologies can map and target human brain with unprecedented precision
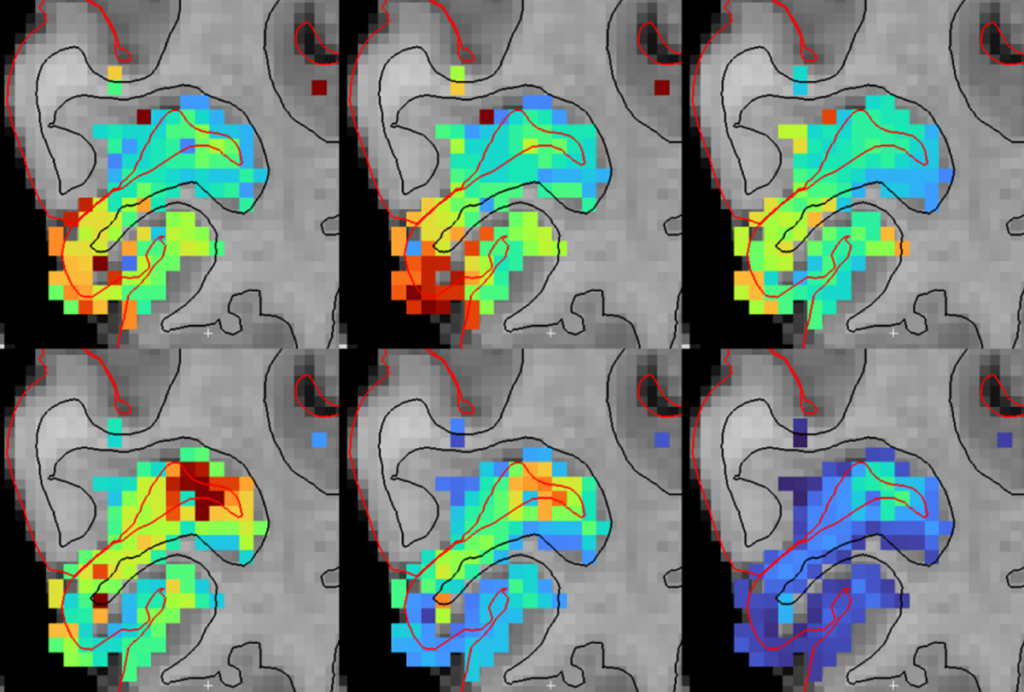
Functional MRI can do more than you think
Explore more from The Transmitter
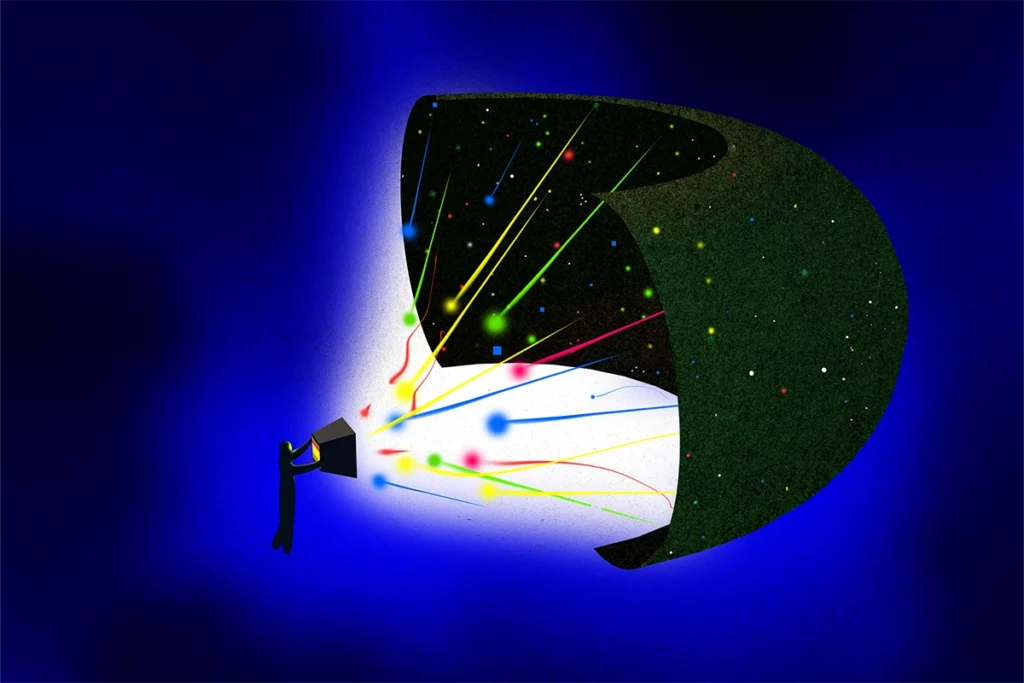
Imagining the ultimate systems neuroscience paper
Expanding set of viral tools targets almost any brain cell type
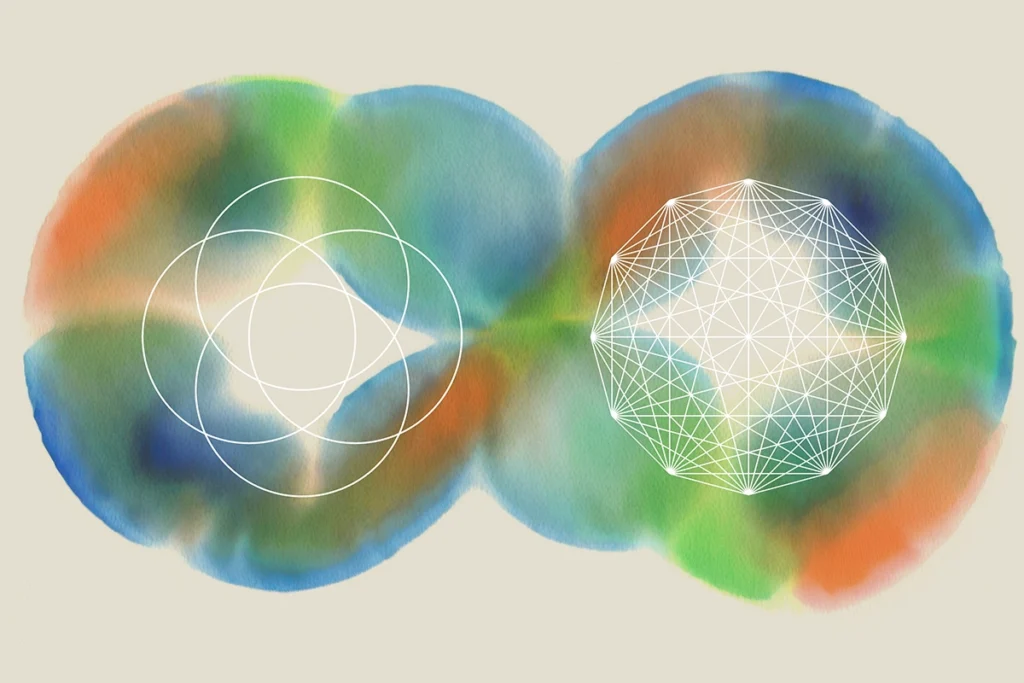