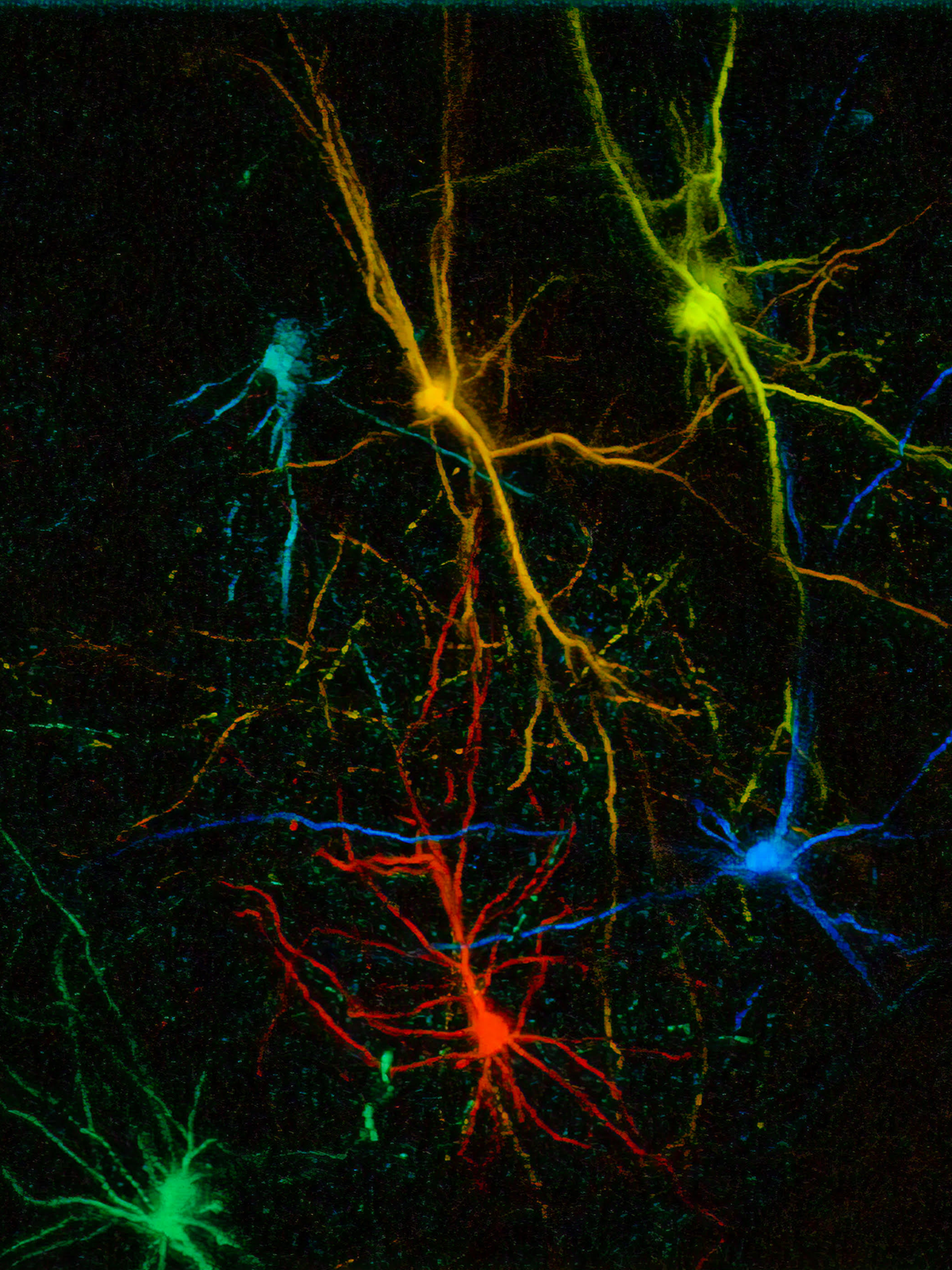
In vivo veritas: Xenotransplantation can help us study the development and function of human neurons in a living brain
Transplanted cells offer insight into human-specific properties, such as a lengthy cortical development and sensitivity to neurodevelopmental and neurodegenerative disease.
Homo sapiens’ enhanced cognitive abilities originate from specialized properties of the human brain. These properties are thought to be the result of evolutionary changes at the level of neural circuits, neurons, glia and synapses. Beyond brain evolution, understanding human neural cell development is essential for deciphering diseases. The human species is particularly sensitive to cognitive problems, including neurodegeneration, intellectual disability and autism, that can result from changes in neuronal maturation or function.
Despite the importance of human-specific features of neurons, we know little about how thse cells develop and function within circuits, among other things. That’s largely because human neurons are so challenging to study. But technological revolutions over the past decade are overcoming that roadblock, leading to novel forms of “human neurobiology.” Methods to differentiate human pluripotent stem cells (PSCs) into neurons represent one key milestone, enabling researchers to generate specific neural cell populations in a fairly versatile and reproducible fashion.
Xenotransplantation methods, in which human PSC-derived neurons are transplanted into the rodent brain, represent another important step, providing a way to study cultured human neurons in an in-vivo setting. Researchers can then observe and manipulate the development and function of transplanted human neurons within the neural circuits of a living host brain. Although still in its infancy, xenotransplantation has led to substantial progress that would have been difficult or impossible to achieve with animal or in-vitro models alone. Scientists are using the techniques to explore human-specific features of neural development and novel pathogenic mechanisms of neurodevelopmental conditions, as well as to test new therapies.
X
enotransplantation of human neural cells into a nonhuman brain is not a novel technology. Decades ago, researchers grafted human fetal midbrain into the rodent striatum in pioneering experiments that tested cell replacement therapies for Parkinson’s disease. Newer “chimeric” approaches combine PSC-derived neurons and xenotransplantation to study uniquely human neurobiology in vivo.One remarkable feature of human cortical neurons compared with those of nonhuman primates, for example, is their unusually prolonged or neotenic development: Human cortical neurons and circuits take years to fully mature, compared with only a few weeks for mouse neuronal counterparts. This prolonged development is thought to be central to humans’ advanced cognitive and learning abilities, and disruptions to this process might underlie intellectual disability and autism.
Xenotransplantation experiments are shedding light on the mechanisms underlying human cortex neoteny. Cortical neurons made from PSCs derived from humans, mice and nonhuman primates transplanted into the cortex of newborn rodents retain their own species-specific timing of development, in a way that seems to be largely independent of the host brain. Most strikingly, human cortical neurons mature over months to years, even when transplanted into the fast-developing mouse cortex. Despite their protracted development, within 9 to 12 months the transplanted neurons develop complex dendritic arbors and spines and robust patterns of synaptic function and plasticity.
Crucially, the properties of the transplanted neurons are strikingly similar to native neurons in the human developing cortex, validating the physiological relevance of the model. For instance, calcium imaging and optogenetics revealed highly functional neurons; they produce physiological responses tuned to selective sensory stimuli and can influence the transplanted animal’s behavior. These findings thus strongly suggest that the protracted development of transplanted human neurons mimics that of the human brain. They also indicate that the neoteny that characterizes human cortical neurons originates—at least in part—from the cell itself.
Researchers are now using xenotransplantation to search for potential molecular mechanisms underlying neuronal neoteny. Previous research found that human-specific genes, called SRGAP2B/C, can slow synapse development when engineered into mouse neurons of the cerebral cortex. To test their involvement in human neoteny, researchers created SRGAP2B/C-deficient human cortical neurons and transplanted them into mouse brains. These neurons matured much more quickly than their unaltered counterparts, reaching synaptic maturation milestones 12 to 18 months post-transplantation rather than after a few years. These experiments provide a first example of how xenotransplantation can illuminate uniquely human neurobiology, which would not have been possible with animal or in-vitro models alone.
In parallel, xenotransplantation has been used to study mechanisms of brain conditions, in particular intellectual disability and autism. To investigate how variants in the SYNGAP1 gene affect human neuron development in vivo, researchers transplanted PSC-derived neurons that display the same SYNGAP1 gene variants as those found in people diagnosed with intellectual disability and autism. The SYNGAP1-deficient neurons developed synapses more quickly than typical neurons, integrating into the host cortical circuits earlier than expected. As a result, SYNGAP1-deficient neurons transplanted in the visual cortex responded to visual stimuli months ahead of the typical developmental schedule. These data indicate that faster synaptic maturation can lead to precocious functionality within brain circuits, which could in turn alter the early function and plasticity of infant brain circuits. Researchers can now investigate these hypotheses further in experimental and clinical studies.
Finally, xenotransplantation studies led to the surprising finding that SRGAP2B/C and SYNGAP1 act together to control the slow speed of human synapse development. More specifically, a new study revealed that SRGAP2B/C genes are required to maintain normal levels of SYNGAP1 at the synapse of human cortical neurons in vivo. This indicates that species-specific properties of human neurons can modify their sensitivity to pathogenic pathways. Interestingly, a similar conclusion came from xenotransplantation of healthy human cortical neurons into mouse models of Alzheimer disease. The transplanted human neurons were sensitive to the toxicity of host-derived amyloid plaques, leading to prominent pathological features—including neurofibrillary tangles and neuronal necroptosis—that were not detectable in similarly exposed mouse cortical neurons.
Notably, xenotransplantation of glial cells, which likewise display species-specific features, has revealed that human glial cells can confer specific physiological or pathogenic properties to the transplanted brain, thus shedding new light on human-specific glia-neuron interactions.
B
eyond pathogenic mechanisms, xenotransplantation models also provide a promising system for developing new therapies. This is particularly relevant for molecular approaches, such as antisense oligonucleotides (ASOs), for which the efficacy and selectivity of action may vary in different species. A recent study on Timothy syndrome, a neurodevelopmental condition caused by a variant in the CACNA1C gene, provides proof of principle. Researchers made human neural organoids from cells engineered to carry the pathogenic variant and transplanted them into the rat brain. They then treated the animals with a specific ASO targeting the pathogenic part of the gene, rescuing the cellular phenotypes of the affected cells, validating that ASO as a potential treatment.Xenotransplantation can also be used to develop novel cell-replacement therapies, such as the transplantation of inhibitory neurons for epilepsy or oligodendrocytes for myelination diseases.
Despite these recent advances, xenotransplantation has its pitfalls. The technology remains delicate and labor-intensive, and it combines difficult and diverse techniques not often found in the same laboratory. Moreover, the model is intrinsically artificial, leading to the generation of brain circuits often made of neurons of different developmental ages and synapses of different species. Though much work remains to improve the models, xenotransplantation stands out as a promising approach to interrogate human neurons in vivo. Together with innovative animal models, in-vitro PSC-derived tools, ex-vivo human brain tissue cultures and in-vivo human neuron exploration, it is part of a new cocktail of approaches likely leading to exciting discoveries on uniquely human neurobiology and disease mechanisms.
Recommended reading
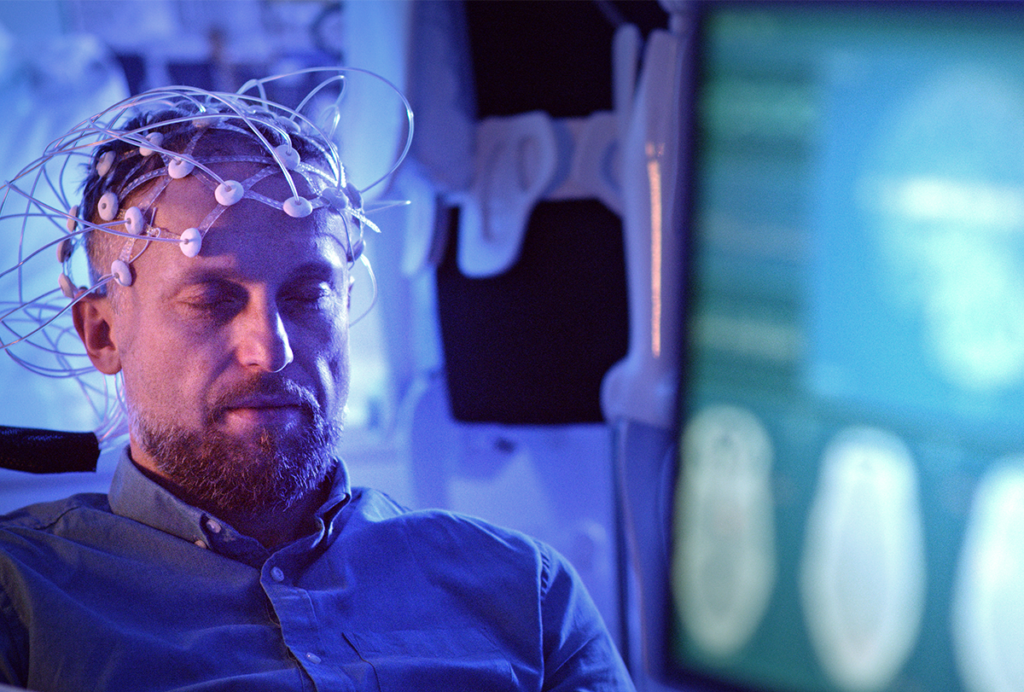
Noninvasive technologies can map and target human brain with unprecedented precision
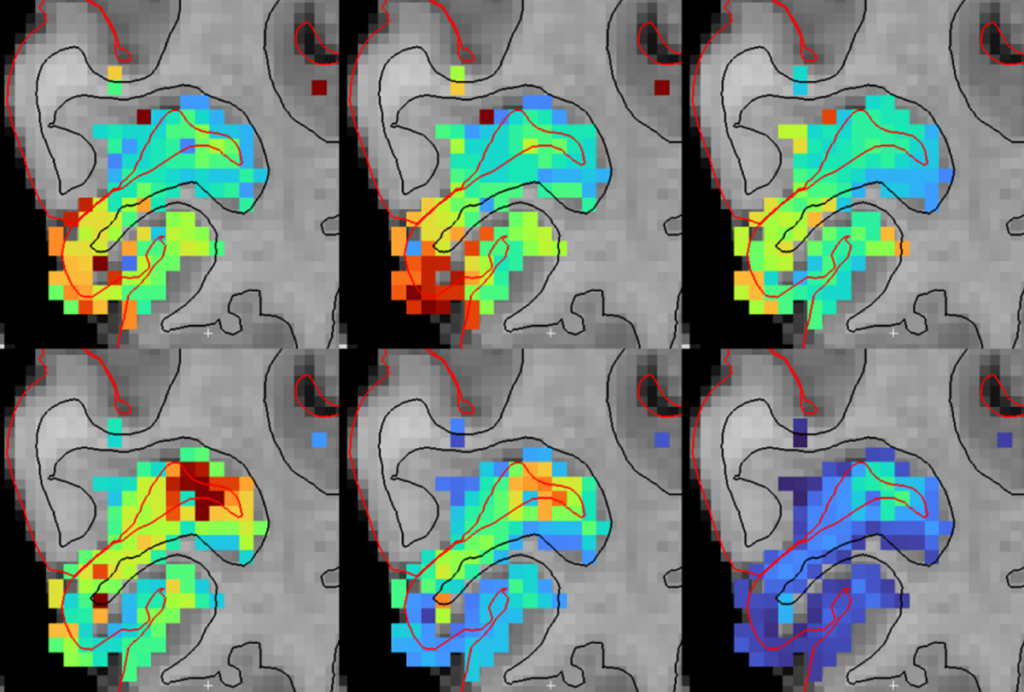
Functional MRI can do more than you think
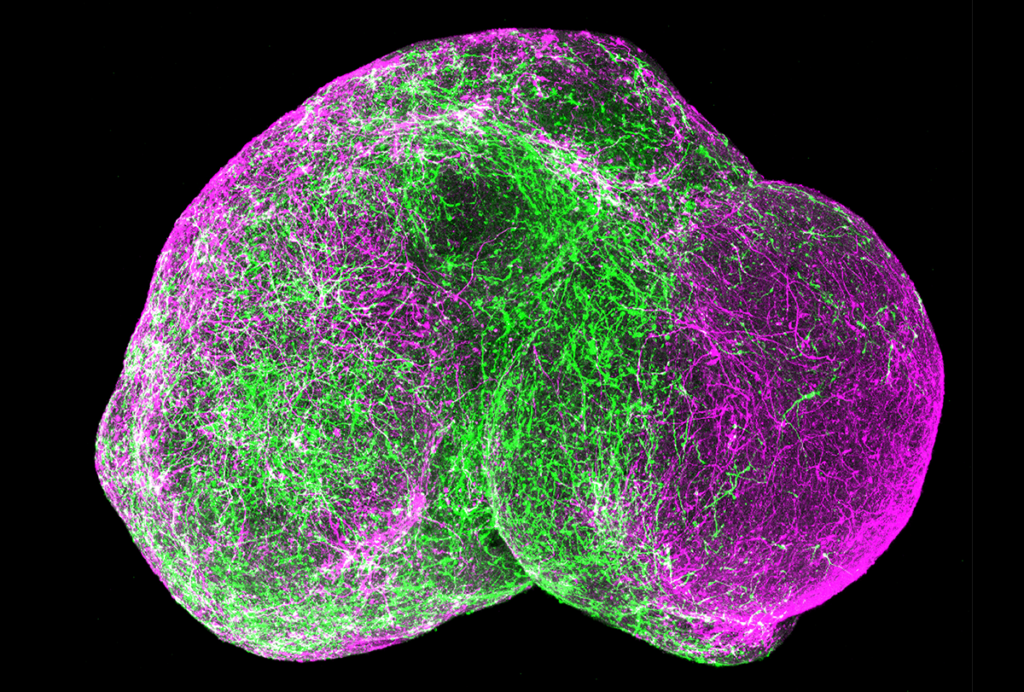
Organoids and assembloids offer a new window into human brain
Explore more from The Transmitter
Expanding set of viral tools targets almost any brain cell type
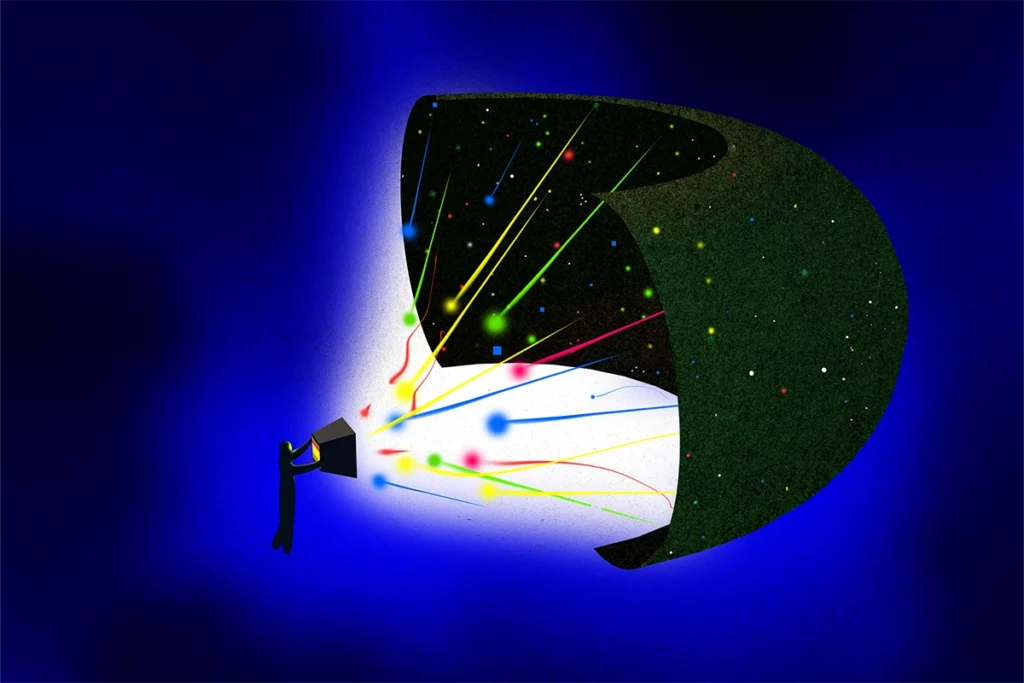
Imagining the ultimate systems neuroscience paper
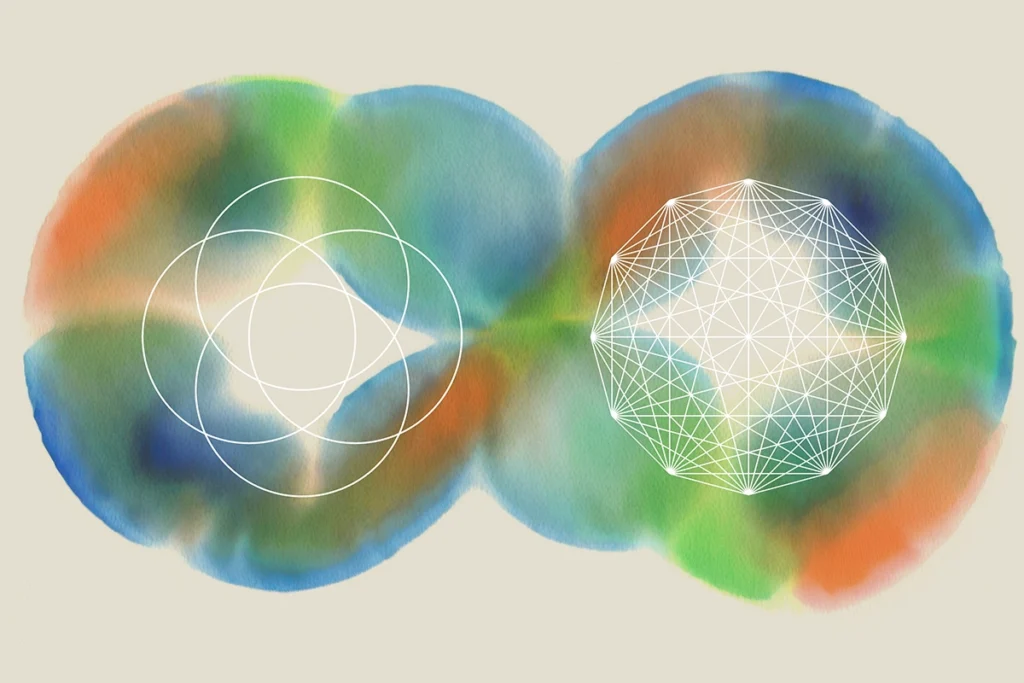