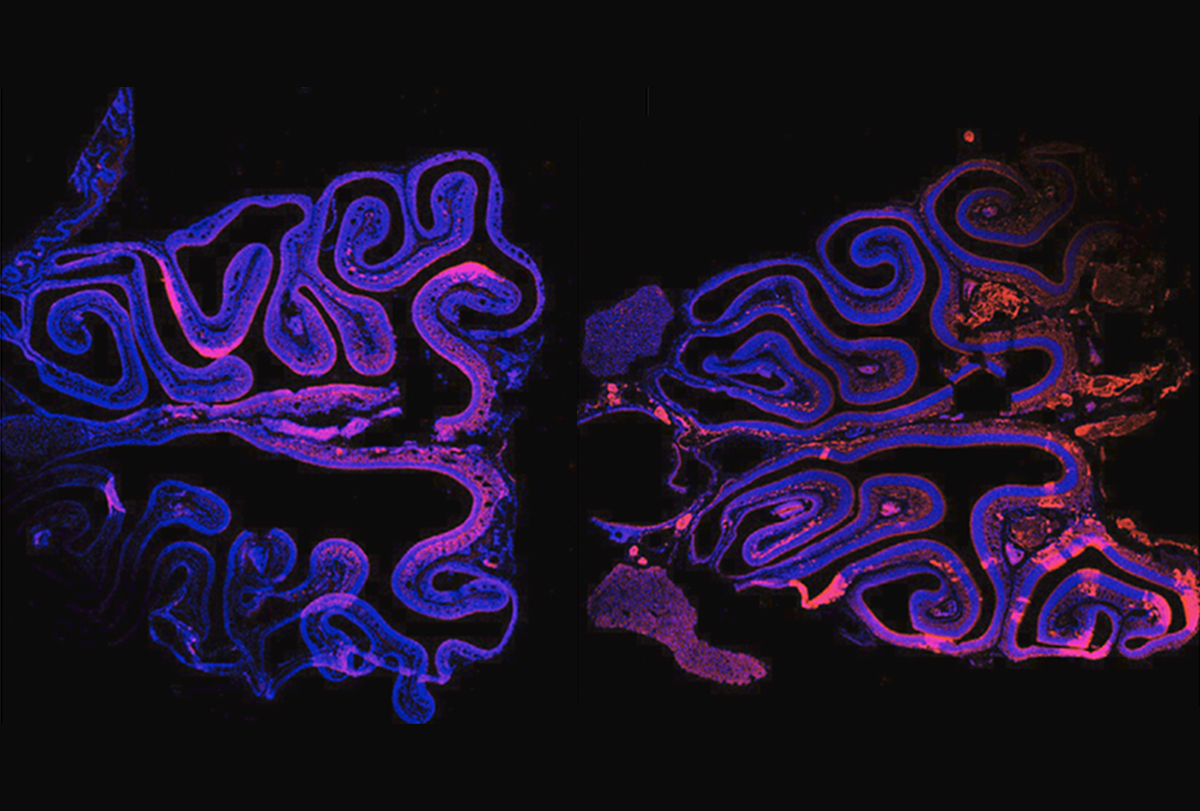
Is it time to worry about brain chimeras?
Brains made of neurons from two species raise new concerns.
As all neuroscientists know, a host of ethical, legal and technical hurdles make it extraordinarily difficult to study the human brain at the cellular and molecular levels. Yet such studies are essential if we are to understand brain function and dysfunction and design novel therapies—especially given the major differences between the human brain and those of more accessible model organisms. How can we move forward?
Over the past decade, several methods have emerged that begin to get around the bottleneck by growing human neurons outside of the human brain. Neural transplants and chimeras, for example, involve introducing human neurons or glia into the brains of nonhuman animals. In transplants, small numbers of neural progenitors or even large aggregates of human neurons, known as organoids, are transferred at late-fetal or postnatal stages. The transplanted cells then differentiate and form reciprocal connections with host cells. In neural chimeras, stem cells are introduced at such an early stage, generally the blastocyst, that they can colonize the entire body, including the brain.
These methods are invaluable given the obstacles to studying the human brain in situ. However, while they circumvent some of the most serious ethical and legal issues, others remain. Accordingly, a few years ago, the National Academy of Sciences convened a committee, which I co-chaired, to survey the current state of the relevant science, examine associated ethical issues and weigh in on whether additional oversight mechanisms might be appropriate.
Many ethical issues came to light, but our overall conclusion was that there was little chance that organoids or chimeras could soon achieve deeply troubling capabilities such as consciousness, emotion or the ability to feel pain. At the same time, we all agreed that the technology is developing so fast that the topic should be revisited in a few years. In particular, we were troubled by the idea that someday researchers could generate a chimeric animal in which the brain of a nonhuman is populated in large part by human neurons.
That still hasn’t happened and is unlikely to happen anytime soon, but two recent papers render it no longer unthinkable. (For more on these studies, see “Rat neurons thrive in a mouse brain world, testing ‘nature versus nurture.’”)
B
oth studies used a method developed more than 30 years ago for generating mouse chimeras. Researchers inject genetically engineered mouse pluripotent embryonic stem cells into a mouse blastocyst, which is then implanted in a female mouse. When the method works well, the result is a “germ-line chimera,” in which some sperm are derived from the donor cells and therefore carry the donor genome. In subsequent generations, mice can be bred to comprise only the donor genome. Until recently, this is how knockout and knockin mice, now mainstays of biology, were generated.Several years ago, researchers modified the method by generating chimeras in which endogenous cortical cells were genetically deleted from the host, resulting in a chimera in which the cortex was composed primarily of donor cells. The new studies used the same strategy but with stem cells from rats rather than mice as donors. Huang et al. deleted host forebrain neurons, while Throesch et al. deleted olfactory sensory neurons, enabling the rat neurons to colonize different regions. The transplanted rat neurons generated appropriate structures and mediated functions associated with the host regions, such as navigation and olfactory-dependent food-seeking, respectively.
Does this imply that a human-mouse brain chimera is possible? Researchers have previously generated chimeras in which human glial progenitors are transplanted into neonatal mice and differentiate into mature glia. They can outcompete mouse astrocytes and, if transplanted into a myelin-deficient mutant, differentiate into myelin-forming oligodendrocytes. But using a similar approach with human neurons instead of glia faces serious technical obstacles—for example, human neurons take far longer to mature than rodent neurons.
Chimeras in which human neurons populate a primate brain are not out of the question, however. Maturation times fall in a similar range, and numerous other factors are better matched. In fact, humans and chimpanzees diverged much more recently, roughly 6 million years ago, than rats and mice, roughly 12 million years ago; humans and macaques are somewhat more distant relatives, with a last common ancestor roughly 26 million years ago. Though creating such a human-primate brain chimera remains infeasible—and is prohibited by a host of regulations and laws—it has become a possibility that we have to face.
I
t’s this scenario that raises the greatest concerns. Unsurprisingly, we feel closer kinship to our primate cousins than to other species that could be used as hosts for transplants or chimeras. As models of human disease improve, hosts may behave in ways that would be considered distressing in people. Genetically engineered macaques and marmosets, for example, show behaviors reminiscent of anxiety and depression. It will be far easier to recognize these behaviors in monkeys than in mice, and we will feel differently about them.Adding to this species issue is the special status of the brain. Researchers have generated human liver and muscle chimeras—human into pig, for example—with minimal controversy. But we are our brain in a way that we are not our liver or kidney. If I receive a kidney transplant from an organ donor, there is little doubt that I would still be me. But if I received a brain transplant, it’s not so clear who I would be.
The reactions to this scenario, from scientists, regulators and the general public, play out on two levels. First are issues related to the status of a human brain in a nonhuman animal. We are fairly confident that human organoids won’t exhibit consciousness or feel pain, but the issue is less clearcut for brain chimeras. What special protections will animal hosts and cell donors need under these circumstances? And how can we conduct these experiments and still maintain our moral duty to be stewards for and respect the status of nonhuman animals?
Second is a sense of squeamishness. Many people view some products of biological experiments as repugnant, especially when they involve the generation of human-like structures. This widely shared feeling of revulsion has been called, inelegantly but evocatively, “the yuck factor.” A highly publicized example was implantation of a polymer-cell template onto the back of a mouse, where it grew into a structure that resembled a child’s outer ear. The possibility that neural transplants and chimeras could generate animals that behave in ways we view as human will likely elicit a similar response, and perhaps lead to the villainization of the researchers. The response will only be amplified by the knowledge that the human-like behaviors are generated by human neurons.
How much weight should we give this feeling? Not unlimited. Looking outside of neuroscience, some practices that were previously viewed as taboo are now widely viewed as ordinary—for example, gay marriage. On the other hand, some prohibitions remain repugnant—for example, eating human flesh. Where should chimeras fit in that spectrum? The conclusion seems to be that feelings of repugnance should trigger serious consideration but not necessarily be decisive.
In addition to these and many other concerns, there is an issue of informed consent. People who have no global objections to neural organoid or chimera research might nonetheless be upset to know that their cells have formed a brain in a monkey. Regulations relating to informed consent have been strengthened greatly over the past few decades—now anyone asked to donate biological material must be fully informed about what will be done with it. Assuming adequate enforcement, new donors can opt out of certain applications. Previously obtained “deidentified” material, however, including cell lines, are not subject to these regulations, meaning the original donors do not have to offer consent for new uses.
Some of these lines are extremely valuable, because they have been extensively characterized over a long period or were derived from donors with rare phenotypes. Clearly no one was thinking about organoids or chimeras when the lines were collected, and some donors might have objected to these applications if they knew. Should their use be allowed? Should consent requirements be different when cells will be used to make brain chimeras than, say, muscle or liver ones?
Given these considerations, what should we do? In many ways these concerns are similar to those raised by other new methods, such as genome editing. Those technologies are overseen by a network of federal regulations and guidelines, as well as institutional committees, such as institutional review boards and institutional animal care and use committees. In the near term, this system seems adequate to oversee chimera research. However, given the rapid advances in the field and the special status of the human brain, this may not be the case for long.
Recommended reading
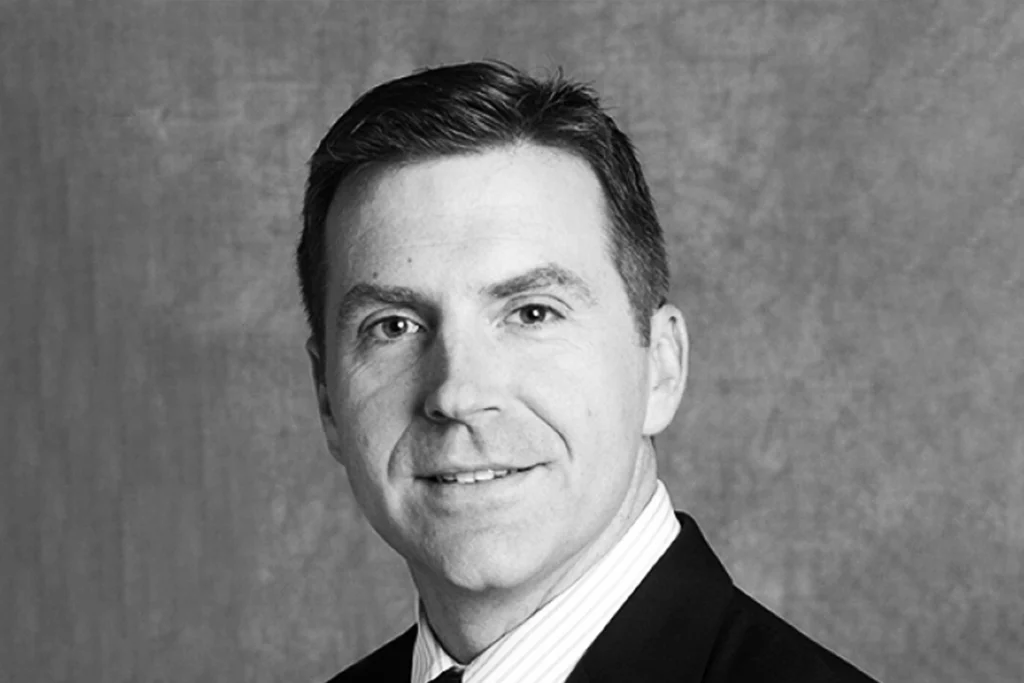
Former Columbia University psychiatrist committed research misconduct, says federal watchdog
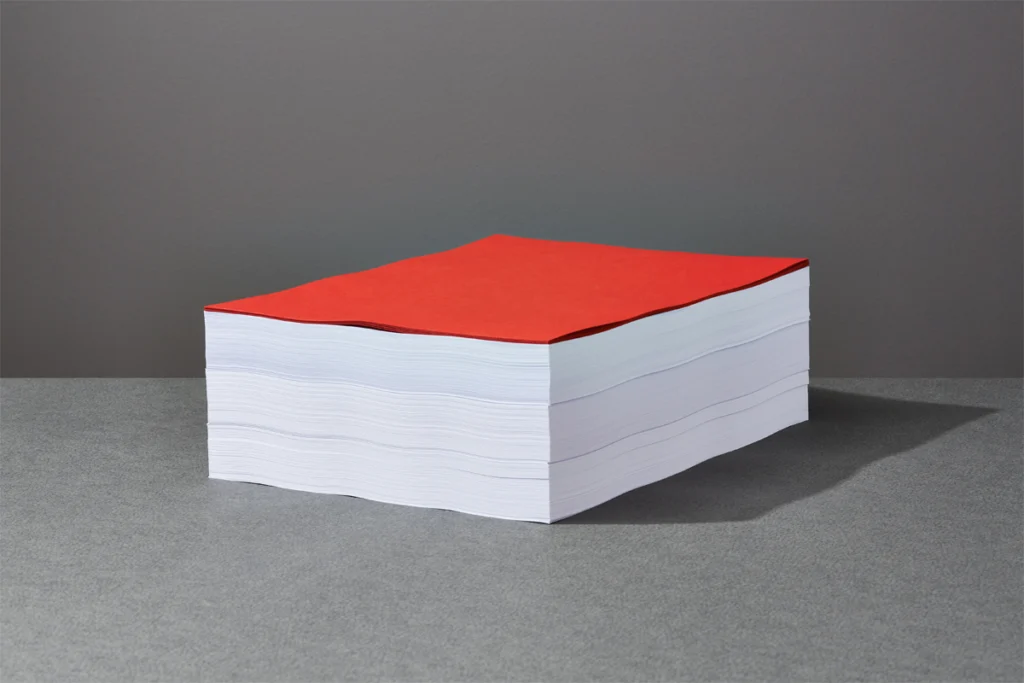
‘Star’ neuroscientist faked data in paper and grant applications, U.S. government finds
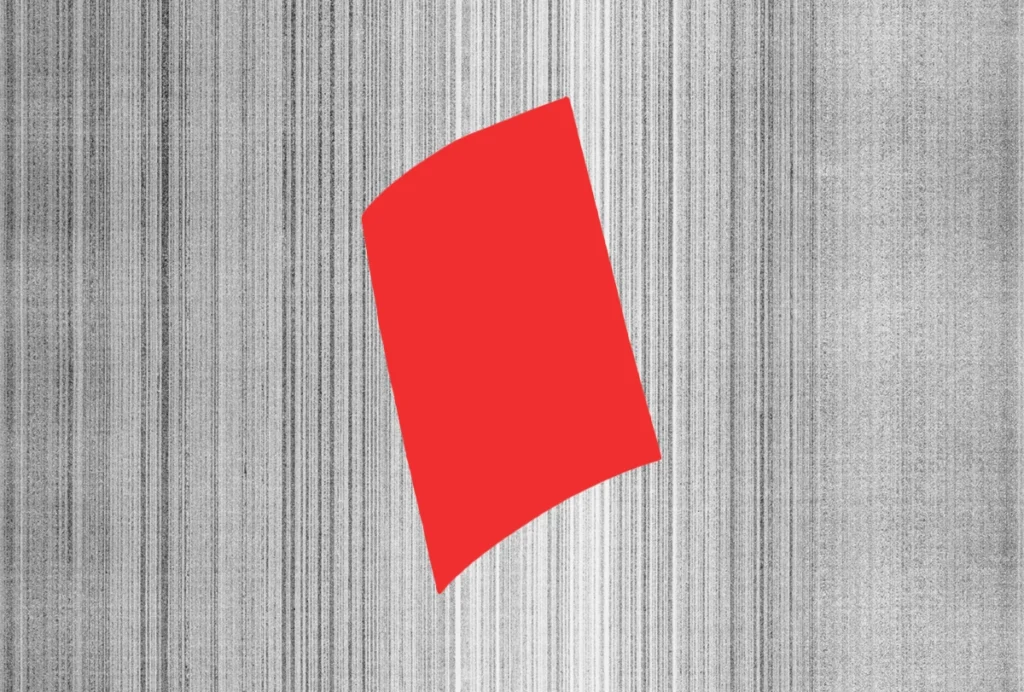
FDA describes ‘objectionable conditions’ at New York State Psychiatric Institute
Explore more from The Transmitter
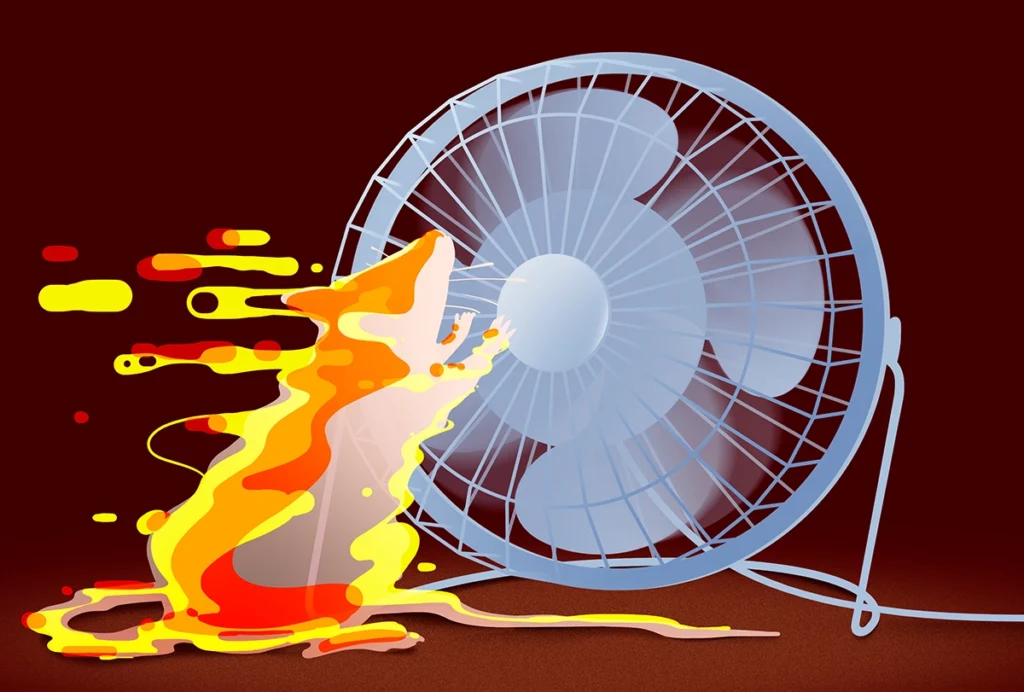