‘Into the wild’: Moving studies of memory and learning out of the lab
People with electrodes embedded deep in their brain are collaborating with a growing posse of plucky researchers to uncover the mysteries of real-world recall.
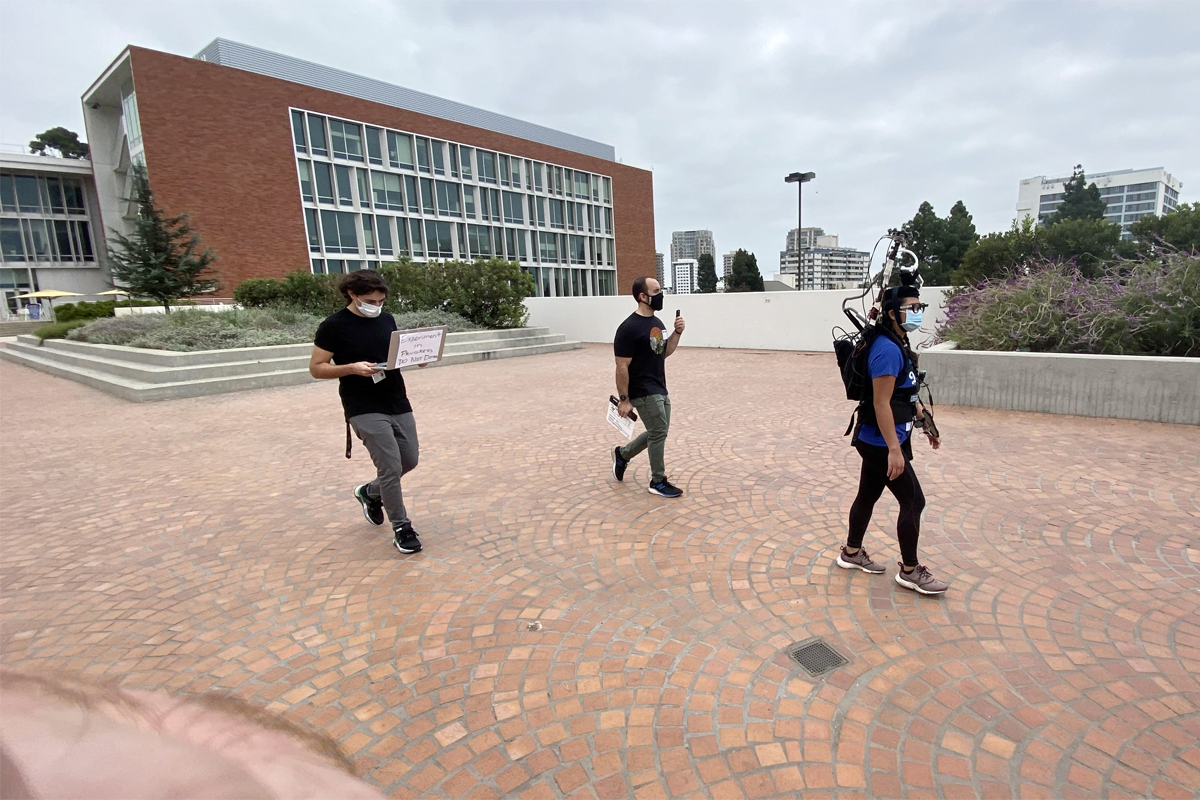
Right at the bench; left at the table and chairs; straight through the sliding doors.
It may sound like a simple set of directions, but there’s no shortage of benches at the University of California, Los Angeles, the setting for one of the most high-tech yet naturalistic studies of human learning and memory to date. Study participants have to learn to navigate a real-world maze across the sprawling campus. They then don wearable devices that track their movements while electrodes implanted deep in their brains capture electrical changes as they log and retrieve memories along the way.
“We don’t make all our memories sitting in front of a computer in a lab,” says study investigator Cory Inman, assistant professor of psychology at the University of Utah in Salt Lake City. Inman became involved in the memory-maze experiment as a postdoctoral researcher in Nanthia Suthana’s lab at the University of California, Los Angeles because, he says, he was eager to move studies of human learning and memory out of the lab and into the wild.
“The memories we make — the ones that really matter to us, that help shape who we are and our identities — those memories are made at the real scales of life: going to the park; going to the aquarium; going skiing with your family or friends,” Inman adds.
Inman and Suthana are part of a small but mighty league of crafty young researchers collaborating with people with epilepsy to unlock the mysteries of memory. About 2,500 people with epilepsy in the United States have devices implanted in their brains that record the electrical signals associated with their seizures and generate signals to disrupt those seizures. As a bonus, the implants are helping researchers answer questions that have been difficult to pursue in the lab: How do our brains discern relevant people and places from irrelevant ones? What is the brain signature of remembering or recognizing something important? And can we improve the brain’s capacity to form, store and recall memories?
Suthana, associate professor of psychiatry, neurosurgery, bioengineering and psychology — and, by all accounts, the crew’s creative captain — was a postdoc studying the role of the hippocampus in learning and memory when an implantable device made by NeuroPace was approved to monitor and treat epilepsy in 2013. “I knew that a lot of these patients had devices put in the hippocampus,” Suthana says. “I thought, ‘This is going to change everything. We can have those people carry out their tasks of daily living and record from this brain area.’ We’ve never been able to do that before.”
In the 1970s, live brain-recording studies in freely moving rats revealed “place cells” in the hippocampus and “grid cells” in the nearby entorhinal cortex — neurons that encode where the animals are in space and help them get where they want to go, like a GPS system and a compass. The work earned John O’Keefe, May-Britt Moser and Edvard Moser the Nobel Prize in Physiology or Medicine in 2014.
But many studies of human memory and navigation had to rely instead on virtual tasks, wherein participants navigate a computer maze or imagine moving through the real world, either from the belly of an MRI scanner or tethered by scalp electrodes to other large machines. “In rodents, the way your brain represents all these aspects of navigation is different when you’re sitting or lying somewhere and doing a virtual task,” says Nick Turk-Browne, professor of psychology and director of the Wu Tsai Institute at Yale University, who is an East Coast member of the “memory-in-the-wild league.” It stood to reason, he says, that human brains might treat these tasks differently, too.
Early studies that tapped implanted electrodes in people were confined to hospital rooms where people were being closely monitored for seizures. The devices, which protruded from the head, helped to pinpoint ground zero of the cacophony of brain activity that leads to a seizure. Modern implants, approved in the past 10 years, are contained within the skull and can record brain activity and stimulate the brain to interrupt seizures. People with these devices can live independently, work, commute and engage daily in myriad other tasks, offering researchers a unique opportunity.
“It’s really a holy-grail kind of technology for studying human learning and memory,” says Turk-Browne. “It’s a natural accident that the hippocampus is both this critical structure for learning and memory and also a very common zone for seizure onsets.”
T
oday’s implants use multiple electrodes in a wire the diameter of an angel hair pasta noodle to measure local field potentials — waves of electrical activity generated by neurons firing nearby. Inman describes the process in terms of walking a microphone around a football stadium. “You’re not going to pick up individual conversations,” he says, comparing the conversations to signals exchanged between individual neurons. “But you’ll be able to tell if someone scored a touchdown.”The devices let Inman and his colleagues listen in on the neural chatter in the hippocampus as someone recognizes a landmark in the campus maze, or a similar one at the University of Utah, or realizes they have lost their way. But linking the brain signals to those moments of recognition — or regret over a missed turn — has been challenging. To help coordinate the two, the researchers use an arsenal of wearable devices, such as GoPro cameras, GPS, pedometers, accelerometers, heart rate monitors and more, to track the participants’ movements — all of which need to be synchronized “down to the millisecond,” Inman says.
He and his team are looking for neural signatures associated with key moments in the maze. They suspect that turns, doorways and other transitions where what comes next is out of view may be prime moments for memory-making. In future work, they want to use the implanted device to stimulate the nearby amygdala during those moments, to see if they can bolster the memory-making process that takes place there. Revving the amygdala, the brain’s center for processing emotions, “is thought to influence what experiences the hippocampus prioritizes to store in long-term memory,” Inman says.
Such research could have implications for people with memory impairments associated with brain injuries or neurological diseases, such as Alzheimer’s disease and even epilepsy.
Ethical considerations abound, though. It’s possible that instead of strengthening memories, stimulation could impair memory formation. It could also induce seizures.
“This requires a lot of care and consent and medical supervision,” Turk-Browne says.
Turk-Browne and his team are testing the effects of stimulating the hippocampus during a seated memory task and hope to eventually test the effects as participants roam, remember and return to certain locations in a room. In a June 2023 preprint, they reported that local field potentials recorded from the hippocampus track with the location, direction and speed of people navigating a room, and some of these representations persist over time.
R
ecording from the brain during sleep and other activities of daily living in familiar environments, as opposed to contrived settings such as a college campus or single room, represents the next level of studying cognition in the wild.“Most navigation happens in familiar spaces, and that’s something that’s really hard to capture,” says Kathryn Graves, investigator on the preprint and a graduate student in Turk-Browne’s lab. She says she hopes to one day record from the hippocampus during sleep, a critical window for memory-making when “spatial patterns are consolidated and maybe replayed.”
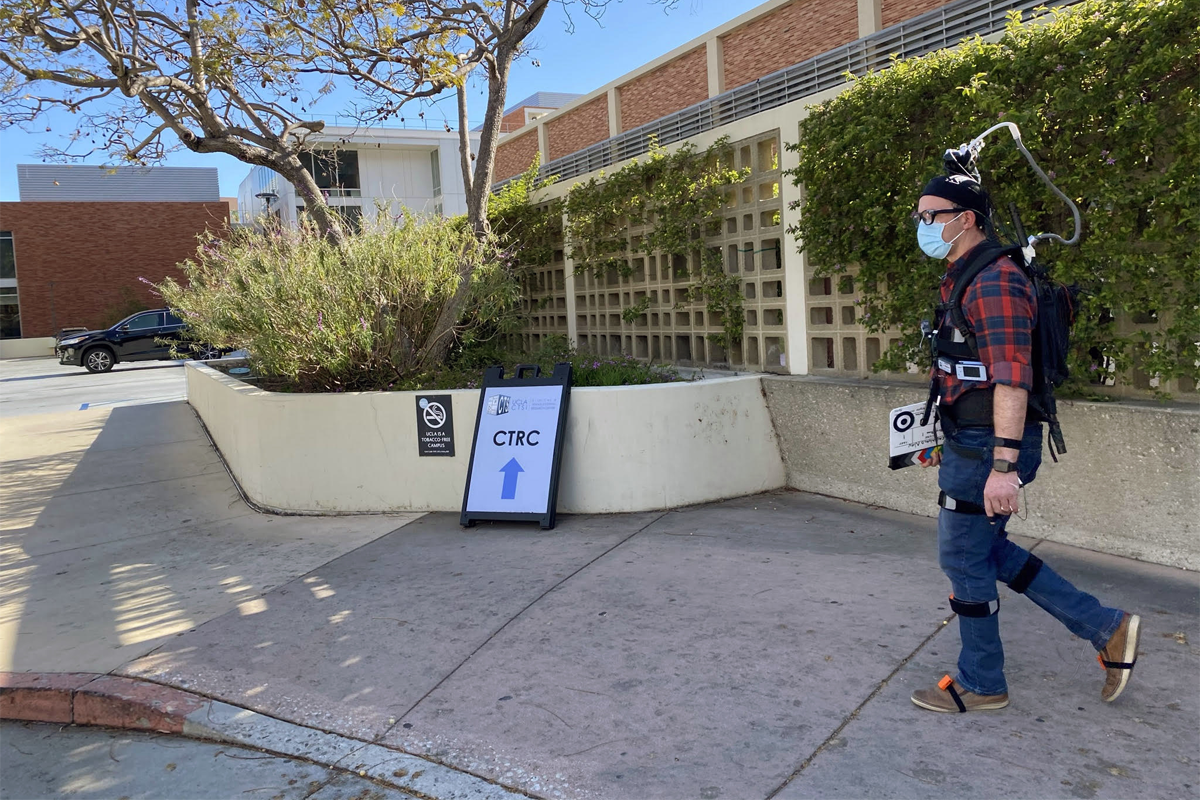
One of the most complex memory tasks that researchers might probe in cooperation with people with implants involves distinguishing an important familiar cue from a similar and still familiar but unimportant one, Turk-Browne says. “I park in a parking garage, and at the end of the day, I need to go find my car,” he explains. “There are 800 parking spots across eight floors. So, I need to remember that today, I parked on the fourth floor on the north side, and I need to distinguish that from everywhere else I’ve parked, including yesterday.”
Moving studies into a participant’s bedroom (or parking garage), though, will require shrinking the wearable tech that can covertly capture their behavior and match it to their relevant brain signatures.
Smaller, more portable devices might also find additional clinical applications. For example, some people with obsessive-compulsive disorder have devices implanted in the thalamus, which abuts the hippocampus. Chronic stimulation is thought to ease their intrusive thoughts and compulsive behaviors — similar to deep brain stimulation for Parkinson’s disease. But overstimulation of the thalamus can lead to hypomania, characterized by decreased sleep, increased impulsivity and general disinhibition. Identifying a brain signature for OCD traits and hypomania, along with a behavioral signature that can be detected through wearables, could pave the way for adaptive brain stimulation that responds to how people are behaving in real time.
“It’s really difficult to elicit these states in the clinic,” says Nicole Provenza, a postdoc in Sameer Sheth’s lab at Baylor College of Medicine in Houston, Texas, about OCD and hypomania. “We need to be doing recordings in the real world, in natural environments, because humans behave in the real world.”
Now that they are outside the lab, Suthana and her “memory-in-the-wild” colleagues see other frontiers, too, beyond spatial navigation and psychiatric disorders, to the study and treatment of movement disorders and even social cognition, according to a review of the field they published in April 2023. “Just imagine you could record from two people with these devices who are interacting,” Suthana says. “Even in animals it’s hard to do that.”
In their latest study, published 20 October in Nature Communications, Suthana and her team showed that brain activity in and around the hippocampus ramps up as study participants approach key locations in a room while performing a virtual-reality memory and navigation task. The brain activity varies with both the goal of the task and the nature of the environment.
“I feel like we’re getting closer to finding out what’s really going on,” she adds. “In the first few years, I felt very frustrated that not many people were excited about this [approach]. Now I feel like there’s a lot of excitement, and I’m excited to see how that translates into more discoveries in other labs.”
Recommended reading
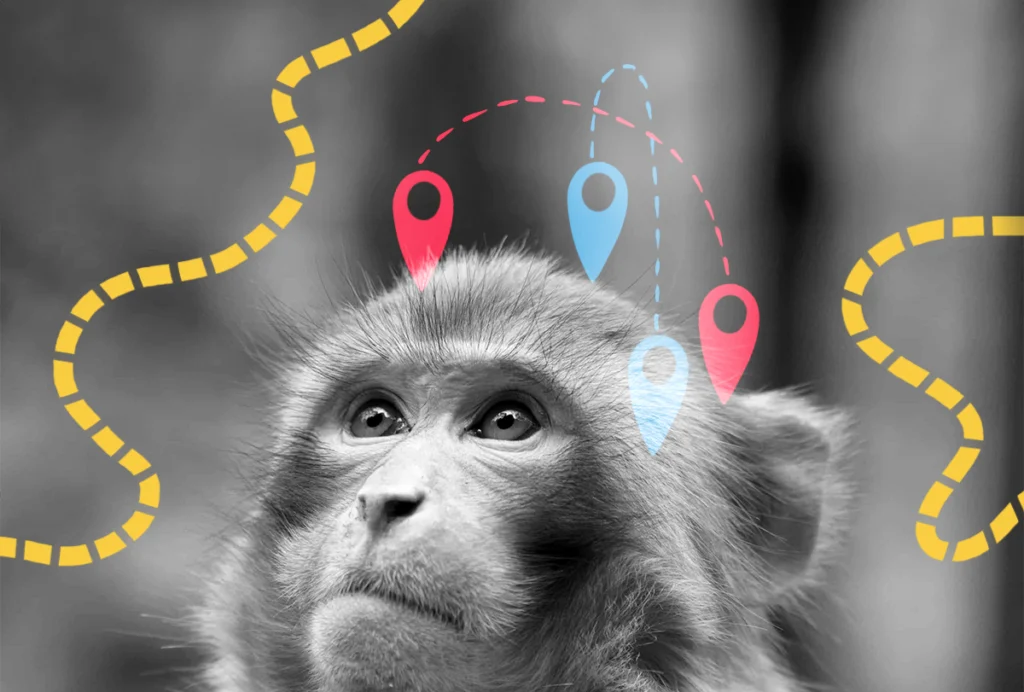
Monkeys build mental maps to navigate new tasks
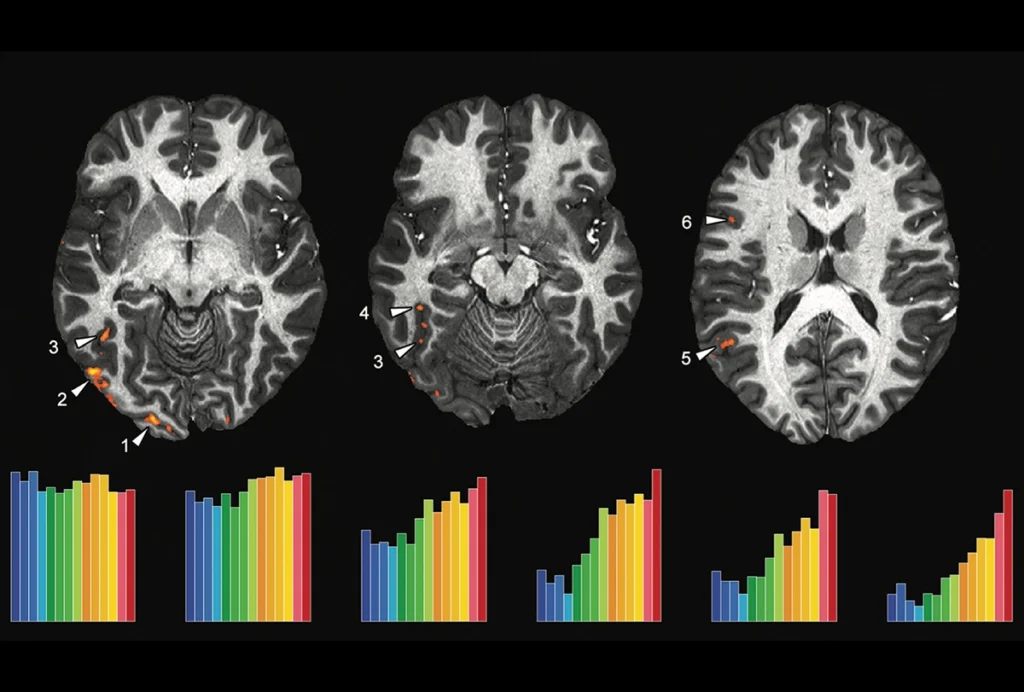
Mathematical brains — an excerpt from ‘Seeing the Mind’
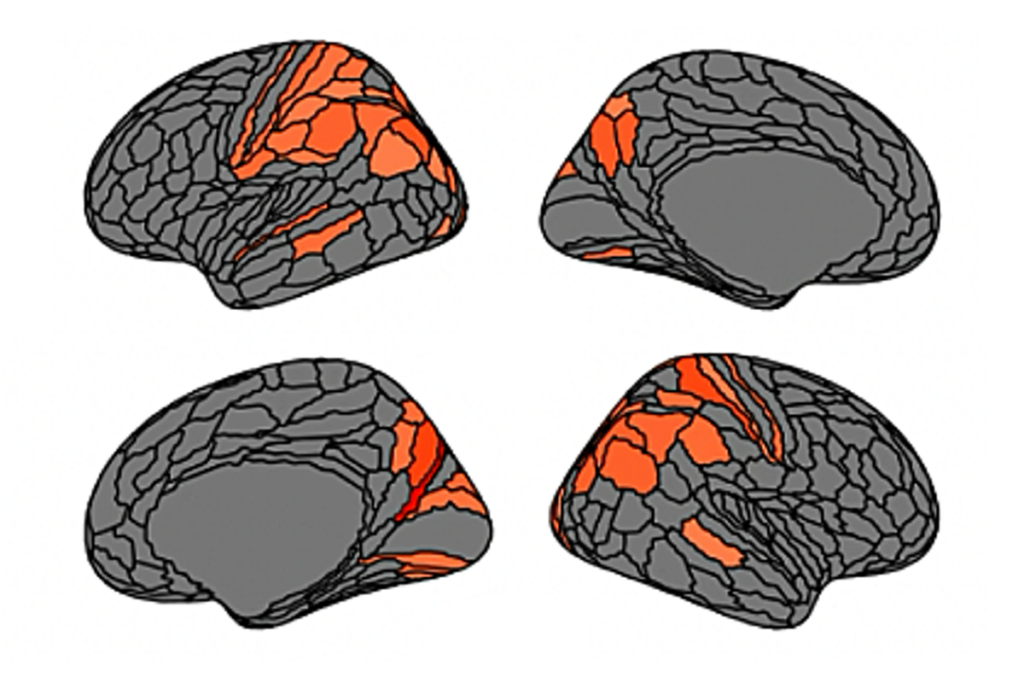
Cortical myelination; early vocabulary; EEG in tuberous sclerosis
Explore more from The Transmitter
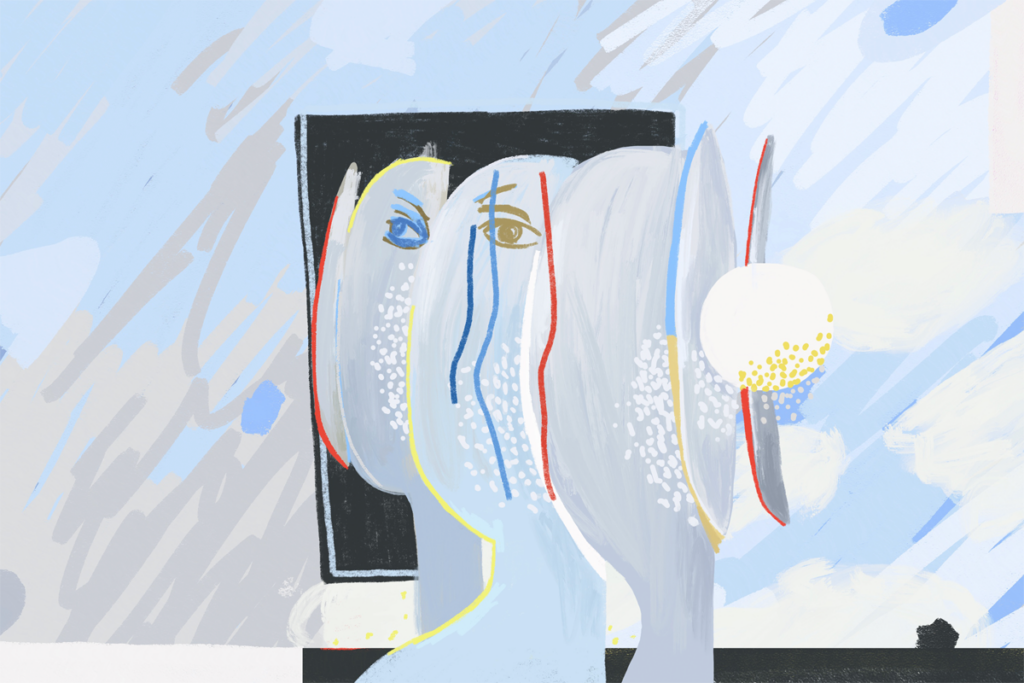
Revisiting sex and gender in the brain
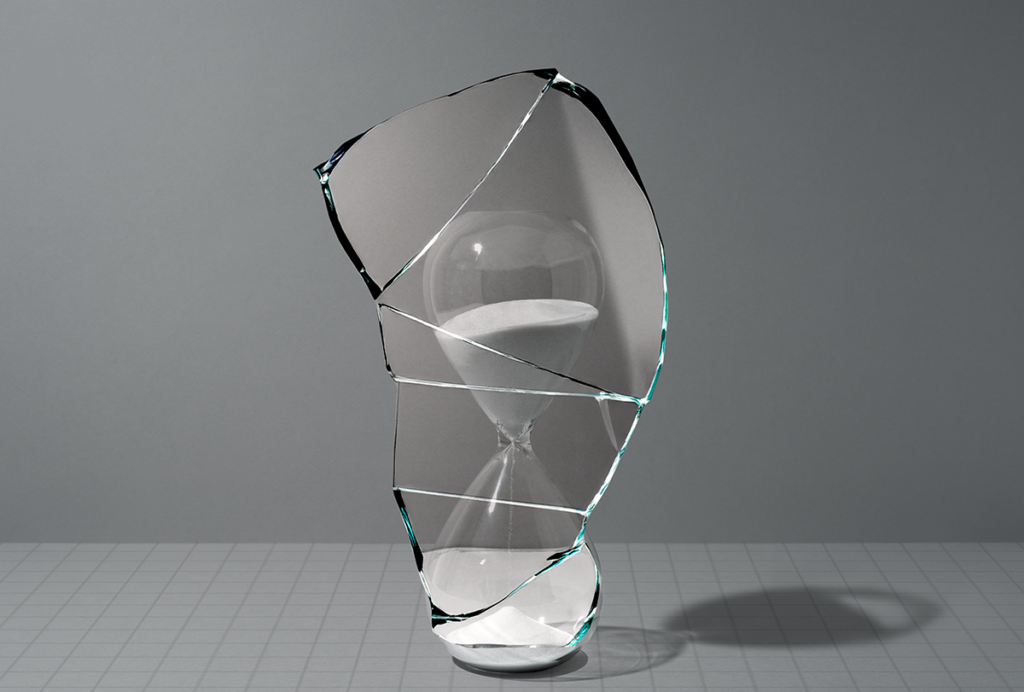
The brain holds no exclusive rights on how to create intelligence
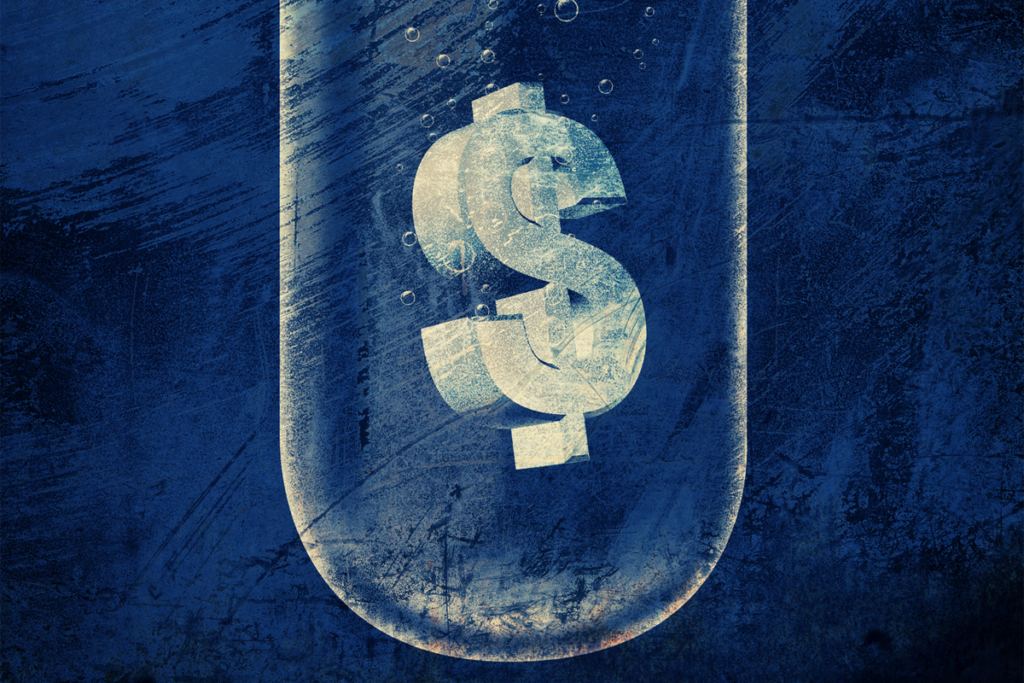